What led you to water analysis and what excites you about it?
Water research, especially water quality, has been a topic of interest for me since my school days. I started with small projects at school, and I knew early on that I wanted to work on something that would help the environment. For a long time, I considered how best to approach this. I thought about more technically oriented study programs but ultimately settled on chemistry, which led me to analytical chemistry – a field I became passionate about right away.
Eventually, I had the opportunity to work on a project that was meaningful to me: developing analytical methods to detect transformation products of nitroaromatic explosives near former ammunition plants. This project also introduced me to the study of contaminants in aquatic systems, especially around ammunition plants, which in Germany date back to WWII. These plants were typically located in areas with abundant water resources, which they needed for production. Even today, water remains one of the most valuable resources for industries, including the chemical industry.
These ammunition plants were a critical source of water contamination, with residues of nitroaromatic explosives lingering for decades after the war. We discovered that degradation products – particularly aromatic amines – were significant contaminants. This work on transformation products naturally led me to investigate the transformation processes of organic contaminants in the environment, particularly in water, and to study how these changes affect water quality, including drinking water.
At that stage, I was focused on chemistry and analytical techniques, but when I did my postdoc in Switzerland, I began to see things from a broader environmental chemistry perspective, thanks to the mentorship of Rene Schwarzenbach, a respected figure in environmental organic chemistry. Working alongside him helped me understand the larger environmental processes at play while continuing to develop analytical methods, especially for polar compounds in water.
At that time, I worked on methyl tertiary-butyl ether (MTBE). When organo-lead compounds were phased out of gasoline, MTBE was introduced as an alternative octane enhancer. However, this substitution was likely made for economic reasons without fully considering its environmental impact. It turned out that MTBE is very persistent in groundwater and difficult to remove with standard treatment methods, which led to public awareness and concern, particularly in California, where signs appeared at gas stations indicating “No MTBE in our gasoline.” This issue might not be as prominent today, but MTBE’s impact remains a reminder of the importance of considering environmental consequences.
From that experience, I began focusing more on polar compounds in water. Given their challenging properties – they are difficult to analyze at low concentrations and can be highly relevant from a water resource management perspective. I’ve continued to work in this field, and it’s safe to say it’s kept me engaged and far from bored.
This issue of substitution; it often seems like we replace a harmful compound with something that has similar properties, only to find that the substitute has environmental or health issues too. Is this a recurring challenge in the field?
Yes, that’s definitely the case. We often need specific properties for certain products, and the compounds that meet these needs tend to bring environmental problems down the line. This has been the story with flame retardants, for example. Flame retardants are essential, as we all know, but every major class of flame retardants we’ve used has also eventually been identified as an environmental concern. We started with organobromine compounds, which became priority pollutants, leading to a phase-out. Then we moved to organophosphorus compounds as replacements, which also bring environmental challenges, especially in terms of how difficult they are to treat once they’re in the ecosystem. You can see this pattern across other areas as well.
PFAS are a particularly challenging example. The stability of the carbon-fluorine bond makes PFAS extremely useful in both industrial and consumer applications. But when these compounds reach the environment, they’re incredibly persistent – something that only started to raise serious concerns about 20 years ago, though they have been in use for much longer.
PFAS don’t degrade easily, so they remain in the environment for decades, if not hundreds of years. These are truly legacy compounds, and they’ll likely stay with us for generations, even if we were to completely phase them out today (which we’re not yet doing, by the way). So yes, these necessary properties often bring unforeseen and lasting environmental issues.
What would you say are currently the most concerning emerging water contaminants?
PFAS are certainly at the top of the list. They’re a major concern, and there’s ongoing discussion across different regulatory bodies about how to best address them. While we now have some threshold values – like those in the drinking water directive – these focus on only a few specific PFAS compounds. The problem is that PFAS as a class is vast and incredibly diverse. There are thousands of PFAS compounds, possibly even millions if we use the expanded OECD definition, which includes any compound with a single CF2 or CF3 moiety. This could extend to most modern pesticides and pharmaceuticals, making PFAS an almost unmanageably large group.
Even with a narrower definition, PFAS compounds vary widely in their properties, so it’s extremely challenging analytically to capture a full picture. That’s why developing a “sum parameter” for all PFAS has been considered – a metric that could give a collective measure of organic fluorine-containing compounds. However, this has proven difficult. There have been some promising attempts that work for certain substances or subclasses, but creating a true, universal sum parameter for all PFAS remains one of the “holy grails” of water analysis. Without it, we’re left with a partial view and can’t be sure if we’re missing significant parts of the contamination profile.
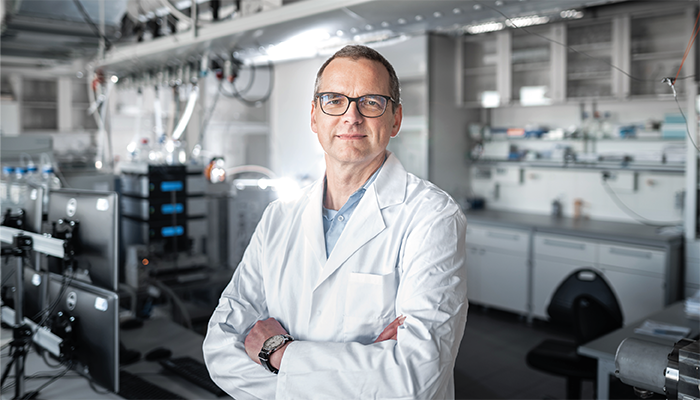
Torsten C. Schmidt
Besides PFAS, are there any other compounds that are similarly concerning or have the potential to become major environmental issues?
Yes. While not a single substance class, compounds that are persistent and mobile (sometimes also toxic), known as PMTs, are increasingly concerning. Some PFAS compounds fall into this category as well. Over the last decade or so, PMTs have gained attention because they have been largely overlooked in the past but are crucial, especially from a water cycle perspective. These compounds don’t degrade easily, and are mobile. They can pass through natural barriers such as soil or bank filtration, and quickly travel through the water cycle. They are very difficult to contain.
Part of the reason they went undetected for so long is because of the limitations in analytical methods, particularly for detecting low concentrations in water. Historically, we relied heavily on gas chromatography (GC) for organic contaminants, which works well for volatile compounds. Later, liquid chromatography-mass spectrometry (LC-MS) became more widespread, but it was primarily based on reverse-phase LC, which doesn’t sufficiently retain highly polar organic compounds. However, advances in recent years have introduced new separation methods that better capture these polar compounds. This progress has revealed that many of these compounds are indeed present, sometimes in high concentrations, in aquatic environments – levels we simply couldn’t detect before.
The growing awareness has been somewhat of a success story, as these persistence and mobility characteristics are now being considered in the registration of new chemicals under regulations such as REACH. This is a positive shift, helping us avoid the pattern of focusing only on certain properties and then discovering new risks decades later.
How much of the full picture are we actually seeing when it comes to contaminants in aquatic environments?
It’s really hard to say, because, as with the iceberg analogy, we don’t know how much lies below the surface until we have the tools to look. Right now, it’s like we’re viewing only the part above water, and we can’t say exactly how deep the issue goes. I suspect that, as methods improve and we start looking for more types of contaminants in ecosystems, we’ll uncover many compounds we aren’t currently aware of.
That said, I don’t think it will expand endlessly. Over the past decades, we’ve built a much better understanding of the types of compounds we’re likely to find. So, while I don’t expect the number of contaminants to suddenly multiply by orders of magnitude, it’s true that the more sensitive our analytical tools become, the more we’ll be able to detect.
At some point, though, we have to ask ourselves about relevance. In academia or scientific circles, it’s valuable to push sensitivity as far as possible, but when it comes to real-world environmental impact, not every detection is necessarily significant. There has to be a balance between finding new contaminants and assessing whether they are actually relevant in aquatic systems.
Torsten C. Schmidt is Professor, Instrumental Analytical Chemistry and Centre for Water and Environmental Research (ZWU), University of Duisburg-Essen, Germany