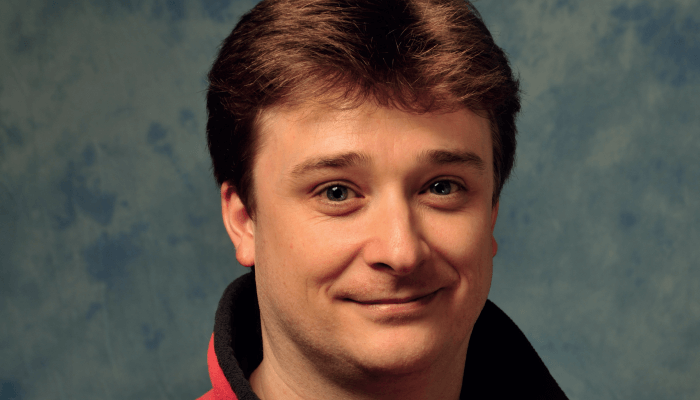
My research focuses on petroleomics: the characterization of petroleum samples and related mixtures using mass spectrometry (MS), particularly ultrahigh resolution mass spectrometry. Petroleum has been called nature’s most complex mixture: crude oil contains organic components represented by tens or hundreds of thousands of different molecular formulae, spanning different functional groups, polarities, and so on. The complexity of this substance presents a range of challenges for the analytical scientist.
Firstly, we must choose appropriate sampling methods: options include taking a one-off sample at a given time, or using passive sampling over an extended period of time (which loses the time resolution of the one-off approach). Next, we must decide on the best methods of sample storage and preparation; particular extraction and fractionation procedures may demand particular solvent and pH conditions, so we must consider the effect of such conditions with respect to the solubilities of each of the huge number of components in our samples. We must choose ionization methods suitable for the sample components, while also considering the potential for fragmentation and generation of different ion types. Similarly, we must select MS approaches according to factors such as resolving power and mass accuracy, and with regard to likely performance when combined with chromatography or MS/MS systems. Finally, we must have access to effective data storage, processing, and analysis techniques; it is crucial to provide outputs that are easy to visualize, interpret, and understand.
For all these reasons, petroleum analysis – just like the analysis of other very complex samples – demands the employment of multiple tools. And that reminds me of the Asian parable of the blind men examining an elephant (the man at the trunk thinks he is touching a snake, the man at the leg thinks he is touching a tree-trunk, and so on). Each man reaches a different conclusion about the nature of what they are examining. In other words, there are limitations to our perceptions and limits to the information provided by any analysis method. We need multiple, complementary perspectives if we are to see the full picture and thus truly understand complex samples.
This multiple-viewpoint philosophy is key to modern methods of petroleum analysis or petroleomics. And it represents a vast improvement on the original methods used for assessment of petroleum-based environmental contamination. Consider analysis of crude oil extracts using negative-ion electrospray ionization techniques; if you rely on that single technique, you’ll primarily observe naphthenic acids but not the many other components of the crude oil. By contrast, the petroleomic approach applies different ionization methods, in both positive- and negative-ion modes, and has thereby identified many problematic compounds other than the naphthenic acids, not least the naphthenic acid fraction compounds (NAFCs).
And so, though I’m convinced of the strengths of Fourier transform ion cyclotron resonance (FTICR) mass spectrometry, especially with regard to mass accuracy and resolving power, I believe that it is essential to use other complementary analytical methods. Relying on a single technique will reveal only one part of our elephant!
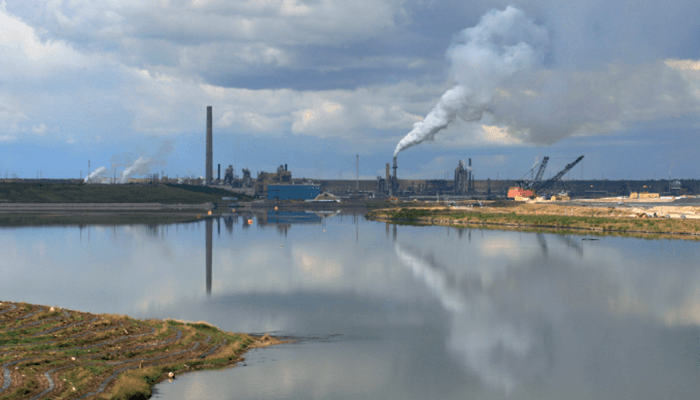
Patterns of success
Petroleomics strategies also assist the analysis of complex samples in fields outside the oil industry. For example, we have been working to improve data analysis and data representation methods for crude oil naphthenic acid analysis – it’s essential to have simple ways of presenting complex messages about highly complex mixtures. We’ve been working with John Headley for many years to apply these same methods to the field of environmental monitoring relating to the Athabasca oil sands in Canada. That’s just one example of how developments in petroleomics can contribute to other fields – there are many others (1).
The broad applicability of petroleomics-related methods is in part a reflection of the fundamental patterns they are designed to recognize – simple patterns that we see time and time again. It’s analogous to the patterns that often underlie the complex forms we see in nature; remember how the Golden Ratio and the Fibonacci series underpin such forms as the nautilus shell and patterns of plant growth. Interestingly, these patterns also find their way into the world of art – Hokusai’s famous print “The Great Wave off Kanagawa” maps onto the Fibonacci series quite closely. I’m often reminded of the phrase “Simplicity is complexity resolved” – which is attributed to Romanian artist Constantin Brâncuși, who attempts to capture the essence of complex forms in his sculptures. Petroleomics has the same philosophy. The analytical tools developed by many researchers within the field essentially help us all to identify common patterns – the petroleomics equivalent of seeing the Fibonacci series – such as differences by H2 (rings and double bonds) or CH2 (alkylation). Common patterns are found both in industrially-derived petroleum and many other samples such as naturally-occurring dissolved organic matter. For this reason, the analytical approaches we employ for crude oil analysis, or modified approaches, can also be applied to environmental samples. After all, such complex mixtures are formed by natural processes, such as the decomposition of plant and animal remains. Of course, there are differences between them too, especially with regard to oxygen content, but the key point is that both types of sample present similar fundamental patterns that lend themselves to comparative analysis.
Real-world impact
Our environmental work includes a collaboration with Environment and Climate Change Canada, spanning nearly two decades, to assess environmental impacts of the oil sands industry in Alberta. These deposits give Canada the third largest oil reserves in the world, but mining the oil sands and refining the oil is very water intensive and environmentally destructive – it demands three barrels of water to produce one barrel of oil, and it has left immense scars on the landscape. Furthermore, under federal regulations, the water used in the extraction process is considered contaminated. It is termed oil sands process-affected water (OSPW) and therefore must not be discharged back into the environment. Instead, the industry stores OSPW in huge man-made lakes called tailings ponds. The water in these ponds is enriched for the molecules that were not retained during the refinement process, such as naphthenic acids. The contamination can actually be visible to the naked eye – you can sometimes see an oily sheen on the water. So advanced methods to analyze OSPW and monitor the environmental impact of the oil sands industry are essential. In one of our papers (2), we compared four different techniques: positive and negative mode atmospheric pressure photoionisation (APPI), which is good for non-polar constituents, and positive- and negative-ion electrospray ionization (ESI). Our results showed that APPI revealed many more peaks than ESI. This further emphasized the point that complex samples contain many components that we won’t see, if we don’t look for them with the right tools.
Historically, however, monitoring environmental contamination in this region is complicated by the fact that the rivers and lakes are in contact with the oil sands, so when you analyze them, you get a complex signature which looks almost like that of a crude oil. How can you tell if the compounds you see have their origin in industrial processes? We have worked on approaches to address this problem, and developed methods that demonstrate clear differences between environmentally-sourced and industrially-sourced water samples (3). They have unique fingerprints. Furthermore, we also demonstrated that it was possible to differentiate between the origins of samples from different companies according to their signatures, and we summarized this using principal component analysis (PCA). In particular, our analytical approach showed clear ratio differences with regard to sulfur-containing species, depending upon the sample origins. Thus, our petroleomics methods not only reveal differences between environmentally- and industrially-sourced samples, but go further and can also differentiate between one industrial source and another.
Since our earlier work, we have been very pleased to see there has been increased use of ultrahigh resolution MS for environmental monitoring in Canada and we continue to work with Canadian collaborators to develop better analytical methodologies. The aim is to truly understand the nature of these complex samples, and to further explore how sample collection, preparation, and analytical technologies may influence the character of a given sample. The latter insight is important; if we are to compare results from different laboratories, it is essential to understand how changes in analytical parameters can affect results. For example, we recently reported that analysis of the O2:O4 compound class ratio (i.e. ratio of CcHhO2 and CcHhO4 compounds) by different labs does not reliably indicate the source of contamination (4). Why? It can be challenging to compare data because labs that use liquid chromatography may change the sample pH to make it compatible with the column and a change in pH can drastically alter the O2:O4 ratio – or even invert it! Our work in this area has emphasized the importance of true, like-for-like comparisons when analyzing and comparing data from different sources.
The methods developed by us and by other researchers help us to better characterize industrially- and environmentally-sourced water samples from the Athabasca region, identify origins, and improve environmental monitoring. Furthermore, improved analytical methods support development of more sophisticated remediation strategies. Remember, the long-term aim is to decontaminate the tailings ponds, so that the water can be returned to the environment. To do that, we must first know what contaminants are in that water, then develop methods to remove them, and finally have reliable techniques to confirm that decontamination has been effective. Robust analytical methods also allow us to answer questions about nature’s involvement. For example, do plants remove contaminants and process them, or do they just store them such that when the plant dies the contaminants are released back into the water? In brief, achieving a better understanding of the range of contaminants assists with both monitoring and future remediation.
We have also compared environmental monitoring methodologies as part of a collaboration with the British Geological Survey (BGS) to analyze soil core samples from Staten Island in the US. As part of the study, we compared two different methods: direct infusion, high field FTICR MS versus lower field FTICR combined with gas chromatography (GC) (5). Interestingly, we also explored how 2-omega detection gives you the option of halving your experiment time for a given resolution or doubling your resolution for a given length of operation. That’s useful when you have a GC step; you want the FTICR’s scan times to be as fast as possible, as the FTICR is typically much slower than the GC.
As analytical scientists, we must be relentless in our pursuit of new methodologies and in our efforts to improve our data analysis – there is always more information to be extracted from a a given (complex) sample. Once we have developed better tools, we can use them in many different applications and so many fields can benefit from advances made during the course of petroleomics research.
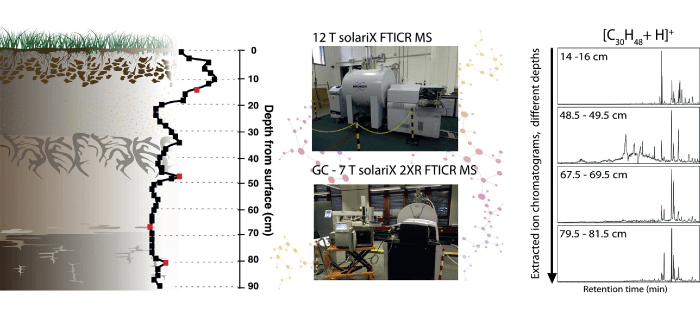
Is there an elephant in the room?
It is clear that ultrahigh resolution MS provides significantly deeper insights into highly complex mixtures. It has helped us establish sample profiles that in turn enable us to better understand both industrial processes. Advanced methods are essential when it comes to understanding the (complex) changes that oil may undergo in the environment, such as through evaporation, exposure to sunlight, and biodegradation. Effective environmental protection strategies need these data to identify and monitor contamination; for tracking contaminants that subtly change over time, we need even more sophisticated methods.
With respect to petroleomics, the ability to analyze very detailed signatures with very high resolution is essential; as I mentioned, each sample contains a huge range of diverse components – polar and non-polar, basic and acidic, large and small. As part of our contribution to advancing analytical methodologies for complex samples, we recently developed a combined experimental and data processing approach, named OCULAR for short, that led to the successful characterization of a particularly challenging non-distillable fraction of heavy petroleum. This resulted in the assignment of a record breaking 244,779 unique molecular formulae – not peaks – within a single sample without the aid of fragmentation or chromatography (multiple peaks may be observed for each molecular formula when using chromatography, due to the presence of isomers) (6). When considering this sort of number of unique molecular formulae and then allowing for the presence of isomers per formula, it is clear that researchers are potentially faced with millions of different structures within petroleum samples! Notably, the existence of many isomers per component of petroleum directly affects the reactivities (and toxicities) of these components and their abilities to interact with catalysts. This latter point is particularly important: the catalyst compatibilities of different isomers determines the efficiency of sulfur removal from petroleum as part of the production of low sulfur fuels.
By developing analytical methods appropriate for complex samples as daunting as petroleum, we contribute to advances in complex sample analysis in many other fields too – and that makes my work even more rewarding.
Although none of us will individually have the full picture, we are collectively getting a better understanding of those elephants!
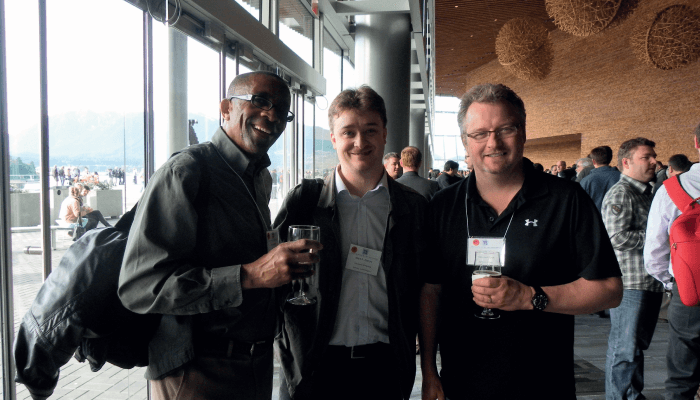
References
- DCP Lozano et al., “Petroleomics: Tools, Challenges, and Developments,” Annual Review of Analytical Chemistry, 13, 405-430 (2020). DOI: 10.1146/annurev-anchem-091619-091824
- MP Barrow et al, "Athabasca oil sands process water: characterization by atmospheric pressure photoionization and electrospray ionization fourier transform ion cyclotron resonance mass spectrometry,” Anal Chem, 82, 3727-3735 (2010). DOI: 10.1021/ac100103y
- JV Headley et al, “Preliminary fingerprinting of Athabasca oil sands polar organics in environmental samples using electrospray ionization Fourier transform ion cyclotron resonance mass spectrometry,” Rapid Commun Mass Spectrom, 25, 1899-1909 (2011). DOI: 10.1002/rcm.5062
- KM Peru et al., “Characterization of oil sands naphthenic acids by negative-ion electrospray ionization mass spectrometry: Influence of acidic versus basic transfer solvent,” Chemosphere, 222, 1017-1024 (2019). DOI: 10.1016/j.chemosphere.2019.01.162
- MJ Thomas et al, “Petroleomic depth profiling of Staten Island salt marsh soil: 2ω detection FTICR MS offers a new solution for the analysis of environmental contaminants,” Sci Total Environ, 662, 852-862 (2019). DOI: 10.1016/j.scitotenv.2019.01.228
- DC Palacio Lozano et al., “Pushing the analytical limits: new insights into complex mixtures using mass spectra segments of constant ultrahigh resolving power,” Chem Sci, 10, 6966-6978 (2019). DOI: 10.1039/c9sc02903f