Keeping Tabs on Dioxins
Exposure to dioxins and polychlorinated biphenyls (PCBs) remains a significant threat to a substantial proportion of the European population. To maintain high levels of vigilance, analytical strategies need to evolve. Here, I propose substantial changes to the EU’s monitoring strategy, changes that channel the power of modern analytical technology to accurately, rapidly and inexpensively monitor food and feed for dioxins, PCBs and as-yet-unidentified toxicants.
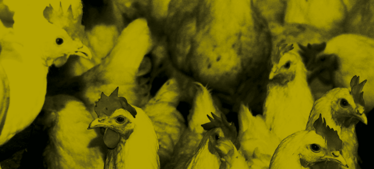
Dioxins first entered public consciousness indirectly. In the late 1950s, chick edema disease appeared: high death rates were reported in broiler chickens in the United States, associated with excessive fluid in the heart cavity, necrosis of the liver, and subcutaneous edema. It transpired that feed manufacturers had been using low-cost fat to increase the energy value of their products and the ‘toxic factors’ responsible for the high levels of chicken mortality were narrowed down to new fatty acid by-products of inedible tallow. It was another decade before 1,2,3,7,8,9-hexachlodibenzo-p-dioxin (which originates from the use of chlorophenols as a preservative in hide-stripping operations) was finally identified as the molecule responsible for the hydropericardium disease (chick edema disease) that killed more than a million chickens (1).
Dioxins and dioxin-like compounds (DLCs) are by-products of various industrial processes, and are commonly regarded as highly toxic compounds that are environmental pollutants and persistent organic pollutants (POPs). The heavy use of Agent Orange during the Vietnam War and Italy’s 1976 Seveso incident raised awareness about the threat of dioxins to human beings. Meanwhile, the Yusho (Japan, 1968) and Yu-Cheng (Taiwan, 1979) food poisoning incidents highlighted the risk of polychlorinated biphenyls (PCBs) – which are precursors of dioxins – entering the food chain. Medical follow-up of the exposed populations in all incidents identified a broad range of severe health effects, some of which were passed onto offspring long after the original exposure (2).
In 1999, although concern over dioxins had faded among the general public (and at health protection agencies), there was another food contamination episode in Belgium (3). The accidental incorporation of contaminated oil (containing about 40-50 kg of PCBs and 1-2 g of dioxins) to a batch of slaughterhouse fat intended for feed production resulted in the distribution of 500 tons of contaminated feed to more than 2,500 poultry and pig farms in Belgium and neighboring countries. This was far from being the largest or most dramatic dioxin-related food incident; however, poor management of the resulting crisis had striking political and economical effects, including some 9,000,000 chickens and 60,000 pigs being destroyed, and an estimated economic cost of €2 billion (4). Fortunately, the general population was not exposed to significant levels of dioxins; even in the highest risk populations, exposure to dioxins barely reached levels that had been routinely observed 10 years before the crisis (5).
The 1999 crisis pushed Belgium to implement maximum residue levels (MRLs) for PCBs in a systematic national monitoring program for food of animal origin (the CONSUM program), and to create the Federal Agency for Food Safety (FAVV-AFSCA) in 2000. This prompted action at the European Union (EU) level, resulting in the implementation of matrix-dependent MRL EU-harmonized norms for dioxins and, later, for dioxin-like (DL)-PCBs. The legislation paved the way for the EU to set up a strategy of continuous monitoring of food and feed in all member states.
The demands of such an ambitious monitoring program required the means and capacity to perform measurements that were cost-effective, precise, accurate and robust. State-of-the-art 13C-labelled isotope dilution gas chromatography coupled to high-resolution mass spectrometry (GC-HRMS) was too expensive to implement on a wide scale. Therefore, a “screening-confirmatory” approach was selected (see Figure 1).
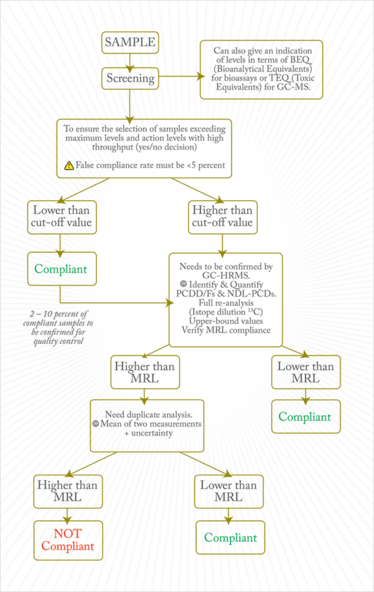
Figure 1. Scheme of the EU screening-confirmatory approach currently in place for the continuous monitoring of food and feed.
Screening uses bioanalytical and GC-MS methods, while GC-HRMS is mandatory for confirmation of suspected non-compliance. Expert working groups proposed strong and detailed analytical guidelines for both GC-(HR)MS and bioanalytical methods to ensure quality. Because it is among the most stable and sensitive assays, the screening method of choice is chemical-activated luciferase gene expression (CALUX) response binding assays (6).
A key aspect of the working groups’ recommendations was the need for a performance-based measurement system (PBMS) that states ‘what’ needs to be accomplished, but not prescriptively ‘how’ it must be carried out. This permitted flexibility in method selection and also allowed for new developments to meet mandated monitoring requirements as improvements of existing procedures became available. Today, 15 years after the introduction of the first post-1999 dioxin crisis EU documents, and after the release of more than 20 major regulations, directives, or recommendations, the PBMS approach is still followed in European Standards (7). The strategy was enhanced by the development of a rapid alert system for food and feed (RASFF), which requires member states to immediately notify the European Commission (EC) about any information on serious health risks related to PCDD/F contamination derived from food or feed.
Improving sample preparation
Food-feed control is far easier to describe than to properly implement. This is because target levels are as low as pico- or femtogram per gram of matrix, with matrix-related interferences present in concentrations at orders of magnitude higher than the analytes of interest. As a result, a multi-step approach is required to (i) extract the analytes from the matrix core, (ii) separate out potentially interfering compounds, and (iii) isolate, separate and quantify analytes of interest.
The clean-up procedure for dioxins in biological samples has its roots in organochlorine pesticide residue analysis. It evolved from single-stage procedures to more advanced multi-steps approaches, such as acid washes and partitioning, silica gel, alumina, and carbon column chromatography, as well as size-exclusion chromatography. In the mid 1980s, due to increasing demands for sample throughput, an automated sample clean-up apparatus was developed for the isolation of 2,3,7,8-tetrachlorodibenzo-p-dioxin from adipose tissues and human serum by the US Centers for Disease Control and Prevention (CDC) (8). Soon thereafter, automated sample clean-up was developed for other bio-accumulative polychlorinated dibenzo-p-dioxins (PCDDs, or dioxins), polychlorinated dibenzofurans (PCDFs, or furans), and ortho unsubstituted (planar, non-ortho) polychlorinated biphenyls (PCBs).
Prior to the clean-up step, several simple variants of preliminary extraction may be used, such as Soxhlet, liquid-liquid, solid-phase, and column extraction. Instrument approaches including microwave-assisted extraction (MAE), pressurized fluid extraction (PFE), and supercritical fluid extraction (SFE) are also popular for the extraction of the lipid portion containing the targeted dioxins.
To date, no “best combination” of extraction and clean-up techniques has been identified. There are several good options, but more than 30 years of investigation have not identified the definitive dioxin sample preparation approach. Thank goodness for PBMS! Essentially any working combination that fulfils regulation criteria can be accepted as a reference method.
Irrespective of the combination used, analysis times are long. This is problematic in the context of food-feed control because foodstuffs cannot stay under inspection for a long period without generating economic losses. More importantly, food safety agencies need fast response strategies to deal efficiently with potential dioxin contamination incidents. Remember, all this is in advance of the measurement itself which, for both biological screening and GC-HRMS confirmatory analysis, will require additional time.
Automation and integration
The proper management of dioxin incidents must be pursued with urgency and effectiveness; this means that reference accredited laboratories must have a certain level of automation. Once alert systems identify a potential issue, the precautionary principle is enforced: this can result in a few thousands farms being blockaded, again with tremendous economic effects. Proper traceability mechanisms and liberation of non-incriminated farms can only start once full analytical data sets become available.
In practice, this means that hundreds of complex sample matrices must be analyzed in matter of days, if not hours. The networking of national reference laboratories ensures widespread geographical analytical capability, while coupling and hyphenation of the various analytical steps ensures rapidity. For example, pressurized liquid extraction (PLE) coupled to automated solvent reduction-exchange devices can produce sample extracts that are further cleaned-up in-line via multi-step liquid chromatography (LC) setups (9). The sorbents used in such LC setups allow the separation of the cleaned extract into subgroups of compounds (PCDDs, PCDFs, PCBs) depending on their polarity and geometry. The fractionated extracts can be further evaporated using a hyphenated solvent reduction-exchange device to reach the required concentration factor, at which point the extracts can be transferred to GC injection vials for separate GC-MS measurements.
Following such approaches, medium-sized laboratories can reach throughput levels of around 20 customer reports per day, including the congener-specific data (on 29 analytes) that are necessary for patterning and source identification. In fact, the current EU confirmatory task force, based in the 27 official or national reference laboratories (OFLs and NRLs), has a combined capacity of approximately 1000 samples per week, which is surprisingly much larger than the screening capacity of 300 samples per week provided by the seven OFLs responsible.
The death of screening?
The tremendous increase in sample throughput afforded by automation and general analytical capacity inside EU laboratories has been mirrored by a reduction in the cost to perform analyses. Today, most food and feed samples can be fully processed, with levels reported on a congener basis, in less than 24 hours (see Figure 2) and for less than €350.
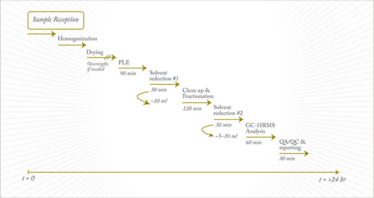
Figure 2.Time line of a typical sample processing procedure for food or feed.
Such confirmatory analysis speed forces us to reconsider the wisdom of following the screening-confirmatory approach. As shown in Figure 3, prices have drastically decreased since the 1999 dioxin crisis era and this trend is expected to continue for the next few years. Perhaps it is time to revise the strategy by using only confirmatory tools to monitor the food-feed web for PCDD/Fs and selected PCBs – this could be renamed the ‘legislative target approach’.
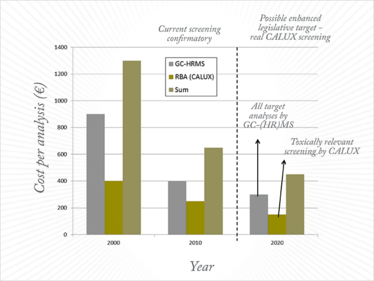
Figure 3. Evolution of the relative costs of screening and confirmatory analyses.
In fact, such an approach was tested in Italy in 2008 (10), when high rates of MRL non-compliant samples were anticipated. In cases where the non-compliance rate exceeds 35 percent, performing the confirmatory analyses exclusively is less expensive than first screening all samples to estimate a global level of contamination and then performing analyses on suspected non-compliant samples. It also reduces the response time and allows congener-specific data to be used to trace the source of contamination and take rapid action. All this can be done at a lower cost than non-congener specific screening analysis in the year 2000.
Given the fact that congener-specific data are a crucial component of the EU’s pro-active approach to reduce the presence of dioxins and DL-PCBs in food and feed, it seems illogical to follow any other path.
Looking five and 10 years ahead
The ‘legislative target approach’, using GC-(HR)MS instruments, would be a cost-effective approach to regulatory analysis of target analytes. Note that ‘HR’ is set in brackets because other types of MS analyzers of the latest generation could also be used. As an example, triple quadrupole systems performing in tandem mode are now also recognized for the official control (confirmatory) at the EU level (11). Such GC-MS analyses benefit from the latest advances in sensitivity enhancement, which is useful given that levels of dioxins are continuously decreasing in food and feed. Tools based on cryogenic zone compression (CZC) should be commercially available in the near future and offer the possibility of boosting chromatographic signals ‘on demand’ to attain low femtogram to high attogram performance levels (12, 13).
This approach removes screening from the equation. However, this does not mean that the CALUX assay ceases to be of value. Instead, it is liberated for broader objectives than simply looking for dioxins. The CALUX response is based on the binding of dioxin-like compounds to the aryl hydrocarbon receptor (AhR); however, CALUX assays are not only sensitive to PCDD/Fs and DL-PCBs, but also to many other AhR agonists, including brominated dioxins and biphenyls, polyaromatic hydrocarbons, benzimidazole drugs, and natural occurring flavones (14). This lack of specificity is a major issue for the legislative use of CALUX as it requires extracts to be processed through several clean-up steps to isolate target analytes from other AhR agonists. Even with these clean-up steps, the use of matrix specific reference samples and cut-off strategies are often required to minimize the risk of false-positive/false-regulatory results.
With the CALUX no longer needing to be ‘tuned’ to satisfy the specific dioxin monitoring program implementation, it can be re-purposed as a real toxicity-screening tool. The CALUX response to extracts is of considerable toxicological relevance. The potential synergistic or antagonistic effects of mixtures of untargeted toxicants is a wide-open question but analyzing high-response CALUX extracts by sensitive GC-MS methods could identify emerging analytes that are capable of triggering an Ah receptor response – in other words, analytes capable of exerting dioxin-like effects. A potential scheme of analysis that uses this is presented in Figure 4.
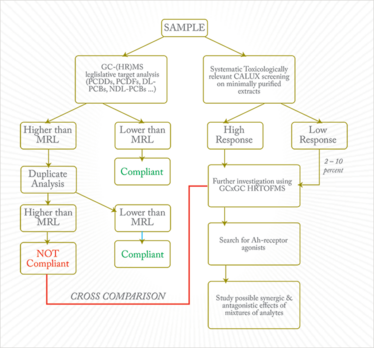
Figure 4. Scheme of a possible new approach for the continuous monitoring of food and feed, as well as screening for other untargeted toxicants.
The selected ion monitoring (SIM) MS scanning mode used for sensitive target analysis cannot be used for the identification of unknown substances; full-scan mass acquisition is needed to screen chromatographic signals for new compounds, and for this the latest generation of time-of-flight (TOF) MS systems has a lot to offer. Current GC-TOF MS instruments offer the mass resolution of sector instruments whilst maintaining limits of detection (LODs) at the low picogram level. The complete deconvoluted mass spectral data set produced by high resolution TOF MS of high mass accuracy can be processed against reference spectra libraries to identify compounds based on fragmentation and exact mass. The high spectral acquisition rates of the last generation of HRTOFMS instruments also allows the chromatographic separation to be operated in comprehensive two-dimensional mode (GC×GC) to enhance both the separation power and compress chromatographic signals for better detectability (15).
The take-home message
Although the EU’s continuous monitoring strategy has been in place for nearly 20 years, I do not consider the dioxin problem to be solved. Levels of dioxins and PCBs in our food web have been decreasing but are still not below the level that would ensure that the entire population is safe. Analytical procedures have evolved, making such measurements more rapid and cost-efficient, to the extent that the current screening-confirmatory approach should be revisited, in my opinion. A legislative target approach not only makes sense from cost and efficiency perspectives, it also frees up CALUX to screen for as-yet-unidentified toxicants responsible for false-regulatory results that could present dioxin-like adverse effects.
A comprehensive strategy that includes legislative target analyses using state-of-the art analytical weapons, coupled to a toxicologically-relevant CALUX screening backed by compound identification tools would more efficiently protect consumers’ health. Taking full advantage of what modern separation science offers will deliver better standards of food safety. In short, we still have some work ahead of us.
Jean-François (Jef) Focant is a professor in the Department of Chemistry at the University of Liège in Belgium.
- D. Firestone, “Etiology of Chick Edema Disease”, Environ. Health Perspect. 5, 59-66 (1973).
- K. Tsukimori, “Long-term Effects of Polychlorinated Biphenyls and Dioxins on Pregnancy Outcomes in Women Affected by the Yusho Incident”, Environ. Health Perspect. 116, 626-630 (2008).
- A. Bernard et al., “Food Contamination by PCBs and Dioxins”, Nature 401, 231-232 (1999).
- A. Covaci et al., “The Belgian PCB/dioxin Crisis—8 Years Later: An Overview”, Environ. Toxicol. Pharmacol 25, 164-170 (2008).
- A. Bernard et al., “The Belgian PCB/Dioxin Incident: Analysis of the Food Chain Contamination and Health Risk Evaluation”, Environ. Res. Section A 88, 1-18 (2002).
- L. Hoogenboom et al., “The CALUX Bioassay: Current Status of its Application to Screening Food and Feed”, TRAC 25, 410-420 (2006).
- CSN EN 16215, Animal Feeding Stuffs - Determination of Dioxins and Dioxin-like PCBs by GC/HRMS and of Indicator PCBs by GC/HRMS, 2012.
- C.R.Jr. Lapeza et al., Automated Apparatus for the Extraction and Enrichment of 2,3,7,8-tetrachlorodibenzo-p-dioxin in Human Adipose Tissue”, Anal.Chem. 58, 713-716 (1986).
- J.-F. Focant et al., “Automated Sample Preparation-fractionation for the Measurement of Dioxins and Related Compounds in Biological Matrices: a Review”, Talanta 63, 1101-1113 (2004).
- M. Esposito et al., “Contamination Levels and Congener Distribution of PCDDs, PCDFs, and Dioxin-like PCBs in Buffalo’s Milk from Caserta Province (Italy)”, Chemosphere 79, 341-348 (2010).
- SANCO/11950R2/2013 to become Commission regulation (EU) No …/.. of…. Amending Regulation (EC) No 152/2009 as regards the determination of levels of dioxins and polychlorinated biphenyls.
- D.G.Jr. Patterson et al., “Cryogenic Zone Compression for the Measurement of Dioxins in Human Serum by Isotope Dilution at the Attogram Level Using Modulated Gas Chromatography Coupled to High Resolution Magnetic Sector Mass Spectrometry”, J. Chromatogr. A 1218, 3274-3281 (2011).
- D. Krumeweide et al., “Zone Compression (t-CZC): A Novel Gas Chromatographic Tool for Increasing Sensitivity”, Chromatogr. Today Feb/March 2012, 19-23.
- R. Hoogenboom, “The Combined Use of the CALUX Bioassay and the HRGC/HRMS Method for the Detection of Novel Dioxin Sources and New Dioxin-like Compounds”, Environ. Sci. & Pollut. Res. 9, 304-306 (2002).
- J.-M.D. Dimandja, “Comprehensive 2-D GC Provides High-performance Separations in Terms of Selectivity, Sensitivity, Speed, and Structure”, Anal. Chem. 76, 167A-174A (2004).
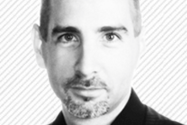
Jean-François (Jef) Focant leads the organic and biological analytical chemistry group of the mass spectrometry laboratory at the University of Liège in Belgium, where his research interests include the development of new strategies in separation science and the implementation of emerging strategies for human biomonitoring and food control. “I’ve been active in the field of dioxin analyses for the last 15 years and chaired the international Dioxin 2011 symposium in Brussels,” says Jef. Well known as a dioxin expert, he is also active in characterization of complex mixtures of volatile organic compounds (VOCs) for medical and forensic applications. “Working on the hyphenation of state-of-the-art analytical techniques to solve practical analytical issues is what I really enjoy doing,” he says.