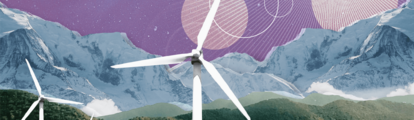
The Complexities of Saving our Planet: For Peat's Sake
Nicholle Bell kickstarts a new series of environmentally-focused articles that shine a light on the researchers putting Earth first
Nicholle Bell | | Longer Read
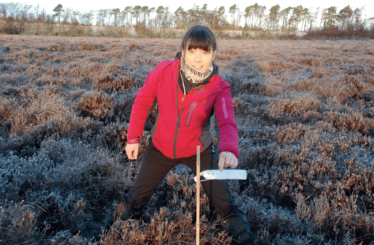
Nicholle, marking each peat core sampling point
It’s a little-known fact that peatlands are the largest store of carbon on the planet. In fact, they store approximately four times the amount of carbon found in all the world’s standing forests, effectively equating to billions of tons. This may sound surprising, but peatland grows at a rate of approximately 1 mm per year, meaning that peat as deep as 11 m – as it is in parts of Scotland – has been accumulating carbon since the last ice age. Why is this important?
Peatlands can only store carbon when they are healthy and wet. And peat draining for agricultural or harvesting reasons opens up the land to oxidation. As you might expect, the result is the release of carbon – either into the atmosphere (in the form of carbon dioxide) or into water systems. For example, if all of the peatlands in Scotland were to become damaged, the release of carbon dioxide would be equivalent to the total carbon emissions of Scotland over the past 140 years. On a wider scale, we would have no hope of fighting the resulting climate-related effects.
Recognizing the importance of healthy peat is the main rationale for my research. I apply analytical techniques, such as high-resolution (HR) nuclear magnetic resonance (NMR), and solid- and liquid-state HRMS to study the chemical composition of peat, which is widely considered the most complex organic mixture on our planet (containing anything between hundreds of thousands to a million of compounds). In particular, my team aims to characterize and understand the differing chemical signatures of pristine and drainage-damaged peat – and whether this change is reversed upon restoration of the land, which is mainly achieved by rewetting the land by blocking drains with plastic dams.
The peat beneath our feet
People tend to think that wetlands (often comprising a fair portion of peat) are effectively wasteland; you can't grow anything because it's too acidic. What’s more, here in Scotland, they’re very cold and there are no trees – so it looks like rolling barren land. There's very little education surrounding them, too, which has resulted in a historical non-appreciation of the incredible work this land conducts right beneath our feet. And when it comes to thinking about how we can meet our climate change targets, the sheer volume of peat in the UK (and elsewhere) makes this a viable target in terms of preventing massive carbon dioxide release.
The UK is number twelve in the list of countries with the most peatland, with approximately 3 million hectares of it. Unsurprisingly, the biggest peatlands in the Northern Hemisphere are in Canada, Scandinavia, and Russia. Then there are tropical peatlands, found in the rainforests of Southeast Asia and other such areas, which contain 30 million hectares of tropical peatland – approximately 10 times that found in the Northern hemisphere. However, this peat is completely different, owing to the differing source of organic matter (largely trees, rather than sphagnum mosses), and the hotter, more humid climate. Matter decomposes more quickly, and the peats are more swamp-like as a result. One of the largest on a global stage is found in the Congo, but it’s rather inaccessible and constitutes a whole other story...
Our process for progress
The good news: our main finding so far from our sites in Scotland is that the same compound classes do return to the peat upon its restoration. When we consider that around 80 percent of the UK’s peatlands are currently damaged – a great cause of concern for our government – that really is good news. But it wasn’t easy for us to arrive at this conclusion.
While we explored the use of HPLC and GC, these techniques failed to separate all the molecules in peat mixtures – as noted above, there were simply too many different molecules. Instead we decided to initial focus on fingerprinting peat samples by compound class, rather than individual molecules. In this regard, NMR is the most effective method – in part because it allows for analysis performance in the solid state, meaning that harsh chemical extractions and the like are not required at the preparation stage. We were able to measure a carbon spectrum immediately from a ball-milled peat sample, and applied a statistical approach to decipher exactly what compound classes were in our samples of damaged, restored and pristine peat.
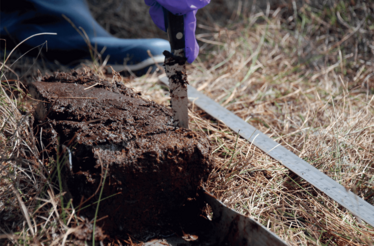
50 cm peat cores are cut into slices to examine changes in the molecular composition with depth
Once we had identified the relevant compound classes, we needed to transition to individual molecule analysis to gain more information – and that demanded alternative analytical routes to the norm. The answer: multidimensional NMR and enriched isotopes. We designed a novel method in which carbon-13 was inserted into molecules in specific locations to replace targeted functional groups; three- and four-dimensional NMR spectra were then allowed us to decipher the chemical structures surrounding the carbon-13 tags (1, 2). After extracting chemical shifts from our tagged samples, we were able to piece them together like a jigsaw puzzle to obtain the compound structures. So far, we have determined the structures of phenolic molecules from peat, but there are many more waiting to be solved.
There were numerous sample prep and workflow considerations involved due to the significant number of confounding factors: peat doesn’t dissolve in anything and molecules often interact with one another in these samples, aggregating and making the analyses increasingly complex. We overcame this by producing a standard protocol, starting with methylation within an organic solvent (a strong base), which results in a large fraction of peat – but, of course, not all of it – in solution.
A toolbox for success
Solid state NMR
- Can obtain information about the carbon compound classes within a peat sample without chemical extraction in tens of minutes.
- Advantage: quick and easy.
- Disadvantage: suffers from spectral overlap, and so cannot be used to determine individual molecules.
Liquid-state NMR
- 3D and 4D NMR experiments (isotope-filtered experiments) were designed to obtain multiple chemical shifts from each tagged molecule, which allowed their structures to be elucidated.
- Advantage: allows unambiguous structural information to be obtained. There are also many NMR tricks one can play to reduce 3D and 4D experiments into their 2D versions.
- Disadvantage: limited sensitivity. These NMR experiments can only determine the structures of major compounds present in the spectra of highly complex mixtures.
FT-ICR MS
- Applied in solid-state (laser desorption ionization) and liquid-state (electrospray ionization) analyses to obtain accurate molecular masses (and hence formulae) for thousands of compounds in peat samples.
- Advantages: sensitivity and resolution.
- Disadvantages: you can only see what is ionizable, and assigning a molecular formula does not equate to a molecular structure.
Onwards and upwards
Now that we have an idea of the compound classes that constitute the difference between damaged and restored peatlands in Scotland, we are aiming to apply our method to peats from elsewhere in the UK, Canada and Sweden to assess the consistency of results across geographies – and to decipher if it is the same specific molecules that are important in each case using our molecular tagging and advanced NMR experiments.
Improving our methods is also a primary concern. At the moment, we take 50 cm cores from areas of peat for analysis; taking peat out of the landscape is not an ideal approach –and we have found an unlikely surrogate for analysis: teabags (3). Teabags represent another complex mixture, and they also contain similar compound classes to peat. Clearly, they are by no means the same compounds, but the classes present are similar enough that we can assess their loss and restoration between pristine, damaged and restored teabags buried in soil across the UK, Canada and Sweden to further add to our understanding of the processes in peat. To this end, we have designed NMR experiments to help us decipher the molecular changes that take place within tea samples (4).
There are many teams working to monitor peatland restoration, but this is often conducted in terms of vegetation, hydrology and gas fluxes – but there have been instances where peatlands thought to been returned to a "healthy" state have yet deteriorated. Such failures really underscore the need for a molecular understanding of the process so that we can assess – with certainty – whether these systems are healthy or not.
In short, there’s a fair way yet to go regarding our work, but advances in chromatography, such as two-dimensional separations and long columns, will make these analyses even more powerful in the future. One of my main motivators in becoming an NMR analyst was the problem solving needed to interpret outcomes and attain structures. Peat, as the most complex mixture on Earth, is therefore one of the biggest jigsaw puzzles on the planet – and one my group is determined to piece together.
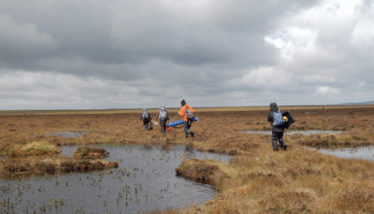
The research team, traversing a peat pool covered with bog bean
- NG Bell et al., “Isotope-filtered 4D NMR spectroscopy for structure determination of humic substances”, Angew Chem Int Edit, 54, 8382 (2015). DOI: 10.1002/anie.201503321
- NG Bell et al., “Isotope-filtered nD NMR spectroscopy of complex mixtures to unravel the molecular structures of phenolic compounds in tagged soil organic matter”, Analyst, 131, 4614 (2016). DOI: 10.1039/c6an00999a
- NG Bell et al., “Molecular level study of hot water extracted green tea buried in soils – a proxy for labile soil organic matter”, Sci Rep, 10, 1484 (2020). DOI: 10.1038/s41598-020-58325-8
- J Sakas & NG Bell, “Reduced dimensionality hyphenated NMR experiments for structure determination of compounds in mixtures”, Faraday Discuss, 218 (2019). DOI: 10.1039/C9FD00008A