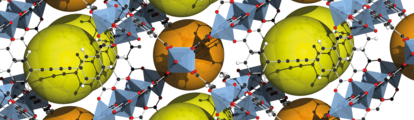
An Underused Framework for Simpler Sample Prep?
Metal–organic frameworks (MOFs) are some of the most promising innovative materials of the moment. Here, I present my “MOF 101” and highlight how almost limitless configurations open up a wealth of opportunities to improve sample preparation.

Structure of BAF-4, with yellow and orange spheres showing the pores. Credit: Tony Boehle.
Sample preparation is typically seen as a necessary evil – and a somewhat neglected field of research. Sorptive extraction techniques, such as solid-phase extraction (SPE) and solid-phase microextraction (SPME), dominate. The evolution of these approaches is mainly driven by the design and synthesis of new materials bearing extraordinary properties – such as metal–organic frameworks (MOFs).
MOFs are crystalline materials with coordination bonds between metal clusters (for example, metal-carboxylate clusters and metal-azolate clusters), metal atoms, or rod-shaped clusters, and multidentate organic linkers with oxygen or nitrogen donors (such as carboxylates, azoles, nitriles, and so on). Within a given material, the length of the organic linker that is used in the synthesis process determines the number of available adsorption sites. Additionally, the characteristic properties of both metal ions and linkers determine the physical properties of MOF networks with regards to their porosity, pore size, and pore surface. The structural properties of the produced frameworks can be controlled by the solvent system, pH, metal-ligand ratio, and temperature (1)(2).
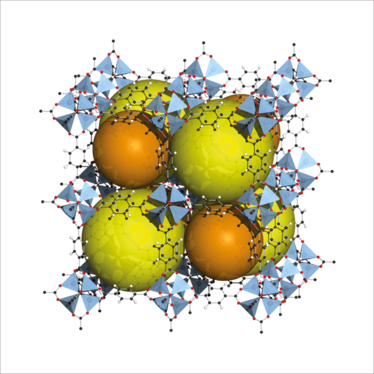
Structure of MOF-5 (also known as IRMOF-1), with yellow and orange spheres showing the pores. Credit: Tony Boehle.
Exceptional properties
MOFs were first introduced in 1990 and their initial uses included catalysis, gas separation, membranes, and electrochemical sensors. In 2006, MOFs were introduced as SPE sorbents for polycyclic aromatic hydrocarbons (PAHs) in environmental water samples. Hydrophobic properties and π–π interaction give them a stronger affinity for aromatic pollutants than conventional sorbents (2)(3).
Since then, their use has expanded further into the field of analytical chemistry, both in chromatographic separation and sample preparation. Analytical chemists have taken advantage of the unique structural characteristics and properties of MOFs in several sample pretreatment approaches, including SPE, dispersive SPE, magnetic solid phase extraction, SPME and stir bar sorptive extraction – with many others likely to be included in the future. The number of analytical applications implementing MOFs as sorbents in sorptive sample preparation approaches continues to increase steadily – mainly owing to the tuneability afforded by the near infinite number of structures that can be designed and synthesized. In the meantime, they have been designed in various formats so that they can meet more challenges with improved analytical features.
MOFs exhibit many interesting characteristics: mechanical resistance, thermal stability up to 300–600°C, cavities of structures with specific pore size, ultralow densities, simple synthetic tuneability, and the highest surface areas (ranging from 200 to 7000 m2/g). And it is these properties that allow them to be used as excellent sorbents in different kinds of analyte extraction (non-polar compounds, polar compounds, and metal ions), such as with pesticides, hormones, antibiotics and phenolics from complex matrices like food, pharmaceuticals, biosamples or environmental samples.
Recently, MOFs have also been applied to the recognition and separation of chiral molecules by incorporating enantio-pure building blocks into the porous framework. Enantio-separation by MOF materials is achieved by interactions between the framework and individual racemic agents, such as hydrogen bonding and the homochiral environment in the pores (4)(5)(6).
In fine form
MOFs are typically classified according to their hydrostability: either moisture-sensitive or water-stable. The instability of moisture-sensitive MOFs in aqueous solution is due to their open metal sites; occupation of those sites by molecular water results in the damage of their structure with a subsequent loss of extraction efficiency. Combining MOFs with other materials can strengthen the stability and dispersibility of moisture-sensitive MOFs in aqueous samples (for example, carbonization, and post-synthetic modification). In this way, their advantages – high surface area, microporosity, and lower density – can still be exploited.
The stability of water-stable MOFs in aqueous matrix makes them compatible for use in SPE, SPME and so on. However, their pore sizes are too small to trap target analytes, thus poor recoveries are observed. Moreover, the hydrophobicity of some water-stable MOFs leads to poor adsorption to hydrophilic compounds. In this case, water-stable MOFs may also need to be functionalized to become more powerful and selective toward target analytes.
To simplify the sample preparation procedure, magnetic composites based on MOFs have also been introduced in magnetic microextraction. These composites, often named after the institution where they were developed, include zeolite imidazolate frameworks (ZIFs), Materials Institute Lavoisier (MIL), University of Oslo (UiO), isoreticular metal frameworks (IRMOF) and Hong Kong University of Science and Technology (HKUST). For more information, see “Know Your MOFs.”
Magnetic MOFs are designed using a composite material with a magnetic center (typically Fe3O4) and a MOF bonded to the outside layer of the magnetic center nanoparticles by means of physical or covalent bonds; for example, via bonding to a polydopamine (PDA) linker (10).
The synthesis of bimetallic MOFs is another approach for synthesizing magnetic MOF materials using a self-magnetic zinc-cobalt MOF with tuned performance by optimizing the ratio between the two metal ions. Other magnetic centers include permanent magnetic materials, such as MFe2O4 (M = Mn, Zn, Ni), which have been recently introduced with various crystal structures and particle sizes (2)(11)(12).
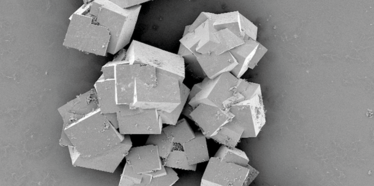
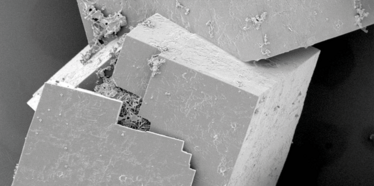
Scanning electron microscope images of MOF crystals. Credit: P Falcaro and D Buso, CSIRO
Know Your MOFs
ZIFs
Constructed by metal atoms (e.g. Zn, Co) linked with imidazolate or functionalized imidazolate ligands through nitrogen atom. They are characterized by high porosity, large surface area, and exceptional thermal and chemical stability either in water or organic solvents (7).
MILs
Built by trivalent metal centers (Cr, Fe) and carboxylic acid bridging ligands. They are characterized by nanoscale porosity, good stability, outstanding surface areas, and availability of in-pore functionality and outer surface modification which make them promising for adsorption and separation (8).
UiO
Built from Zr-based blocks (for example, Zr6O4(OH)4). They are characterized by a high surface area and unexpected chemical and thermal stability (9).
IRMOF
Characterized by a cubic construction with a high surface area (2833 m2/g), great porosity, and easy preparation. They are mainly used in gas adsorption, and are not suitable for aqueous samples because of instability in humid environments.
HKUST-1
3D Cu-based MOF with a big pore size and unique structure. They are especially effective for adsorption of heavy metal ions.
Making a MOF
More “traditional” approaches to MOF preparation include crystallization by slow evaporation (the metal salt and the ligand are mixed in a solution, and crystallization follows solvent evaporation) and diffusion in liquids or gels (the metal and the ligand are dissolved in two separate phases, and the complex is formed when they react after diffusion of one into another). These approaches lead to the formation of large crystals and small amounts of MOFs, and take time – from as little as one day to as long as several weeks.
Modern fabrication of MOFs is based on chemical and electrochemical methods. Chemical production involves solvothermal or hydrothermal processes (depending on the solvent media; the most frequently used organic solvents are dialkyl formamides, alcohols, and pyridine) that synthesize MOFs by means of a cooperative ionic interaction between organic linkers and metal salt in mixed solution. Chemical approaches are comparatively simple and can produce large-scale MOFs, and therefore are preferred in sample preparation applications. The use of microwaves, ultrasounds, or mechano-chemical synthesis speeds up production, and can reduce or eliminate solvents, making the process more environmentally friendly.
In the electrochemical method, MOFs are directly deposited onto a conductive electrode under cathodic potential. In this fast and mild approach, there is no need to add metal salts into the sample solution because they are produced from electrochemical reactions in a solution containing organic ligands and electrolytes. MOFs obtained by electrochemical methods are mainly used in gas separation, membranes, and electrochemical sensors. The use of ionic liquids as either solvent or template has also been applied to the preparation of MOFs and is known as the ionothermal method (1)(13)(14)(15)(16)(17).
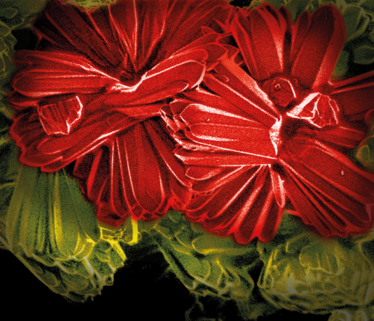
MOF imaged at ×20,000 using a focused ion beam scanning electron microscope. Image from the US Department of Energy.
A promising future
The future applications of MOFs in sample preparation will be determined by the synthesis of new MOFs with improved chemical stability, selectivity, adsorption capacity, and reusability. I expect that a large number of applications using MOF-based sorbents will arise in the near future, because of the almost endless possibilities for synthesizing MOFs and further post-synthetic modifications. When it comes to improving analytical performance, the formation of multifunctional composites – MOF/nanoparticles, MOF/graphene, MOF/silica, and MOF/organic polymers – looks very promising.
Victoria Samanidou is a Professor at the Laboratory of Analytical Chemistry, Department of Chemistry,
Aristotle University of Thessaloniki, Greece.
- B Hashemi et al., Trends Anal Chem, 97, 65–82 (2017).
- X Wang, N Ye, Electrophoresis, 38, 3059–3078 (2017).
- X-Y Zhou et al., J Chromatogr, 1116, 172–178 (2006).
- M Xue et al., Mater Today, 19, 503–515 (2016).
- CL Chang et al., Chem Commun, 51, 3566–3569 (2015).
- X Ma et al., J Chromatogr A, 1537, 1–9 (2018).
- R Banerjee et al., Science, 319, 939–943 (2008).
- RC Huxford et al., Curr Opin Chem Biol, 14, 262–268 (2018).
- JH Cavka et al., J Am Chem Soc, 130, 13850–13851 (2008).
- S Zhang, et al., J Chromatogr, A, 1452, 18–26 (2016).
- Y Wang et al., J Sep Sci, 41, 180–194 (2018).
- C Prestipino et al., Chem Mater, 18, 1337– 1346 (2006).
- P Rocío-Bautista, et al., Trend Anal Chem, 90, 114–134 (2017).
- F Maya et al., Trend Anal Chem, 90, 142–152 (2017).
- ZY Gu et al., Acc Chem Res, 45, 734–745 (2012).
- C Dey et al., Acta Cryst, B70, 3–10 (2014).
- P Rocio-Bautista et al., Anal Chim Acta, 939, 26–41 (2016).
Victoria Samanidou is based at the Laboratory of Analytical Chemistry, Department of Chemistry, Aristotle University of Thessaloniki, Thessaloniki, Greece.