Future Separations
Emily Hilder and Robert Shellie Predict That the Next Wave of Separation Technology Will Be Portable and Personal
Separation science is an enabling science, the fundamental physical processes of which were identified over a century ago (see "Past Separations"). Progress in the field is driven by the demands of biological, pharmaceutical, environmental and forensic scientists, and is realized by developments in technology. Materials science, electronics and engineering are each contributing to the technology truly becoming smaller, faster and smarter.
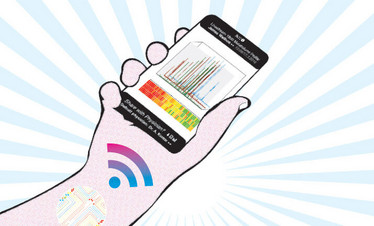
We believe that this progress will continue. In fact, as members of the first generation to grow up with computers, we see the development of computers from large, immovable objects to mobile devices as a model for the development of our field. Over the last 50 years, we have seen a move from classical chromatography to high pressure, high performance systems. API Project no. 6 (see "Classical Chromatography Limits") illustrates the limits of classical chromatography showing the lengths that were required to achieve what is now modest performance using modern systems. In the same way, we expect similar levels of development over the next 50 years, pushing new boundaries. Below we present a snapshot of separation science as it is today, as a prelude to our predictions for future development.
Current separations
As we inch ever closer to theoretical limits, we must ask: “Will chromatography shortly come to a standstill?” To answer this question, let’s first give some brief consideration to the current state of the art.
Evergreen HPLC
High pressure liquid chromatography (HPLC) is one of the most frequently used and relied-upon analytical technologies in the chemical and pharmaceutical industries today, with reversed-phase HPLC at the top of the heap. To achieve this, a great deal more has been invested into enhancing the efficiency of instrument design and in the synthesis of sorbent materials with smaller particle sizes than in new selective materials. Ultra high-pressure pumps and the development of small particles that can withstand such pressure give some UHPLC practitioners the ability to achieve separations with GC-like efficiency (see Figure 1).
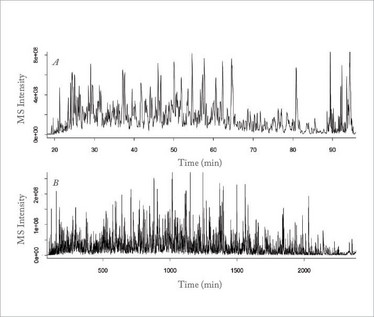
Fig1: Base peak chromatograms for the analysis of E. coli. cell lysate using a 15cm silica particle packed column (A) and a 350cm long monolithic silica C18 column (B). Reproduced with permission (see ref. 1).
Meanwhile, numerous significant developments in materials science have given us both core shell particle technology and monolithic columns (see Figure 2), both of which have led to improved separation efficiency using relatively low pressures. Notwithstanding the heavy focus on efficiency, one of the outstanding features of HPLC is still the incredible diversity of stationary phase chemistries that can be synthesized, which provide real control of separation selectivity. Reversed phase HPLC has been, and will remain, the workhorse of LC, but new developments in stationary phase chemistry, for example, hydrophilic interaction chromatography (HILIC), have extended its reach into exciting new areas such as metabolomics.
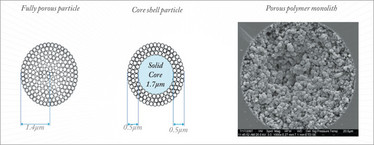
Fig 2: Comparison of different types of stationary phases commonly used in LC
Steadfast GC
Analytical gas chromatography (GC) is an indispensible tool in the petroleum and chemical industries. Widely viewed as a mature technology, it relies heavily on the use of wall-coated open-tubular fused silica and, to a lesser extent, metal capillary columns using convection ovens. Most users rely on high plate number rather than subtle selectivity manipulation to achieve separation of complex mixtures, and development of new stationary phase materials is relatively stagnant. Innovative materials development is often reserved for specific applications; for example, columns for separation of polycyclic aromatic hydrocarbons appeared recently. The recent introduction of a new class of ionic liquid stationary phase is also an area to watch as niche separations are developed using these materials. Analysis and characterization of biodiesel blends is a case in point, where ionic liquid stationary phases provide unique selectivity advantages.
A decade ago, the focus of column R&D was on bleed; the war against bleed is largely over (1 pA over the range from 40 to 300 C). Currently, a focus on reducing column activity is leading to emergence of highly inert capillary columns enabling specific analyses at trace levels that were hitherto impossible (3). Further development will likely broaden applicability to a wider range of polar solutes like amines and diols. Despite the speed and efficiency advantages of using narrow-bore capillary columns, there are relatively few commercial offerings of sub 0.1 mm internal diameter capillaries today and further developments should also be expected in this area.
Miniaturization
Recently, progress in micro-machined instrumentation has been buoyed by the maturation of microfabrication technologies (see Figure 3). These instruments offer smaller footprints, lower energy demands, ultra-fast analysis, and high throughput. Most importantly, they open the door to portable instrumentation, alleviating critical sampling and sample-integrity challenges. Micro-machined sample inlets and detectors will couple with separation columns of smaller internal diameter. Most of these miniaturized systems rely on resistive column heating; a new application of an old technology. Moving away from the convection oven has been a major step towards miniaturization.
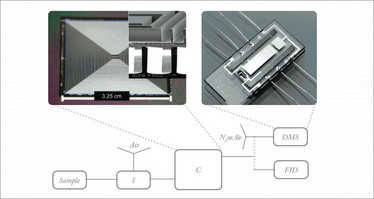
Fig 3: Example of micro-machined instrumentation using a gas chromatography platform. I: split inlet, FID: flame ionization detector, C: microfabricated column (photo inset), DMS: microfabricated DMS cell (photo inset). Reproduced with permission (see ref. 2).
There have also been exciting developments in detection technologies for miniature GC. Two notable examples are the chemiresistor detector developed at the University of Michigan (4) and the use of miniature photoionization (normally used as standalone sensors) in GC detection by researchers at the University of York (5). Miniaturized mass spectrometry is also experiencing a burst of research and development interest.
Multidimensional progress
Access to a wider range of HPLC stationary phases has given us multidimensional chromatography, which uses the unique selectivity of each dimension to maximize separation power. Without such developments, ‘omics’ – in particular, proteomics and metabolomics and related medical research – would simply not be where it is today.
There is also growing interest in multidimensional GC (MDGC) separations. The recent introduction of planar microfluidic column connections, which meet important requirements for MDGC interfacing such as low dead volume, a high degree of inertness for reactive solutes, and efficient sample transfer, has made the approach more amenable. However, data handling, although vastly improved recently, continues to be a bottleneck.
Looking ahead
The separation science pioneers of the past were largely botanists and biochemists. As noted by Richard Synge in his 1952 Nobel Lecture on partition chromatography:
“And here of course the method is giving to the biochemist something akin to what the microscope gave to early biologists […] When the effects with small molecules are better understood we shall be much better able to understand from the structure of the larger molecules what is their function in terms of such intermolecular forces. Then chemistry really will begin to merge with biology as closely as it has already merged with physics. Partition chromatography may well be remembered for this contribution long after it is quite obsolete as an analytical method.”
We see the importance of separation science as a tool in the life sciences continuing, even strengthening. The demand to understand biology in terms of chemistry has only increased. It is also clear that partition chromatography is far from obsolete as an analytical method, as technological improvements have overcome many of the initial drawbacks.
As always, future developments will be strongly driven by the demands of end-users. And, while the underlying demands have not changed greatly, advances in technology have led to much greater expectations. To meet these, separation science should focus on:
- The ability to separate more species in reasonable time frames
- The ability to handle increasingly large amounts of data
- Achieving highly selective and sensitive analysis of specific targets
- Smaller, cheaper, portable and, perhaps, personalized analytical systems
Dimension X
The ability to separate more and more species as we continue to probe complex biological systems will remain a critical challenge. We believe that this will be best addressed through multidimensional separation approaches.
Mature techniques, such as GCxGC and LCxLC, will continue to be developed and refined. Other combinations, with the potential to re-define how we view multidimensional separations, will also appear on the horizon. Two-dimensional combinations, such as LCxGC, LCxCE and CExCE, have already been explored and the proof-of-concept for three-dimensional approaches (GCxGCxGC, LCxLCxLC) has been demonstrated (6, 7). It is reasonable to think that we will soon move even beyond these, with the number of coupled dimensions limited only by our own imaginations. At the same time, this unlimited dimensional progress highlights the great challenge to such systems: handling the sea of data produced.
At a recent meeting of the Australian and New Zealand Society for Mass Spectrometry, we separation scientists were challenged by mass spectrometrists to justify why sophisticated separation technology is still necessary for many applications. The program at the upcoming HPLC meeting in Amsterdam in June 2013 promises to challenge chromatographers with similar questions. We argue that separation science will be more critical than ever. The widespread availability and speed of technological development in mass spectrometry has expanded the range of detection options for separation science beyond what many would have believed possible. At the same time, it is well known that one of the critical limitations of mass spectrometry is its susceptibility to matrix effects; sample preparation or pre-treatment is a key factor in reducing this. The development of highly specific and selective separation phases offers the opportunity to realize the true power of MS technology for the targeted analysis of complex samples, leading to the next generation of multidimensional analysis.
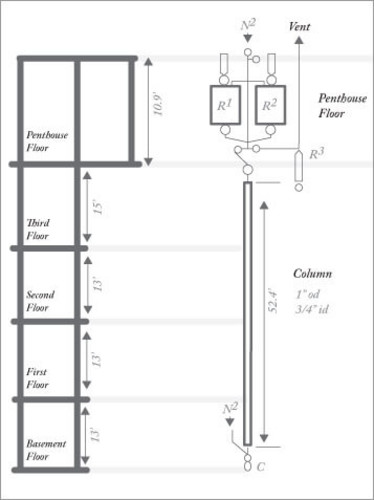
Classical Chromatography Limits
In 1927, the American Petroleum Institute established Research Project No. 6 at the National Bureau of Standards to identify chemical constituents of petroleum fractions. In 1960, F. D. Rossini, project director at that time, estimated that the project had consumed around 500 man-years of work as the system moved from systematic distillation through simple adsorptive filtration to true chromatographic separation. The project was terminated in 1967.
16 m adsorption column (25.4 mm stainless steel tube containing 3.7 kg silica gel, insulated with electric resistance heating wire) housed in 18.9 m shaft. Reservoir R1 holds sample, R2 holds desorbing liquid (alcohol). Typical sample volume: 500 ml. Receiver C collects fractions. Entire system can be placed under inert gas (nitrogen) pressure from top or bottom. Desorbing liquid regenerated by heating column to 200 ºC and collecting evaporated alcohol in receiver R3.
Personalized analytical systems
In our view, the most exciting and significant developments in separation science will stem from miniaturization. Truly portable, and eventually personal, analytical technology is where we are headed.
Developments in ‘lab-on-a-chip’ and similar miniaturization technologies have not yet provided the outcomes that have been promised. The underlying technology, for example, in fabrication and electronics, has been one contributing factor. As fabrication technology develops and improves, we are confident that technologically more ‘difficult’ approaches, such as shear-driven chromatography and microfabricated columns, will emerge as practical alternatives in separation science.
Opportunities to develop from existing portable technology are among the most exciting to us, although they are admittedly far from commercial reality. The number of mobile phone subscriptions worldwide has increased from one to six billion within the last 12 years; this means that 75 percent of the world’s population now has access to a mobile phone, and many of these devices have progressed well beyond simple voice communication to become sophisticated, personalized data handling tools. Using mobile phones for chemical sensing is already possible and with the current rapid pace of developments in mobile technology, it is likely that the impact of smartphones will be felt in separation science sooner rather than later.
In the immediate future, some of the factors driving progress in separation science are far more pragmatic. There is a continuing conflict between pushing a technique to its theoretical limits for maximum performance and the commercial desire for a quick return on investment (8). Certainly, with the numerous demands of the life sciences, as absolute limits are reached, new approaches will need to be explored.
You want to learn more about past separations? Keep on reading.
- M. Iwasaki et al., "One-Dimensional Capillary Liquid Chromatographic Separation Coupled with Tandem Mass Spectrometry Unveils the Escherichia coli Proteome on a Microarray Scale", Analytical Chemistry, 82, 2616-2620 (2010).
- G. R. Lambertus et al., "Silicon Microfabricated Column with Microfabricated Differential Mobility Spectrometer for GC Analysis of Volatile Organic Compounds", Analytical Chemistry, 77, 7563-7571 (2005).
- J. Luong et al., “Chromatographic Sensitivity and Selectivity Improvements for Polar Solutes with Advanced Capillary Column, Capillary Flow Technology and Multi-Mode Inlet” at the 35th International Symposium on Capillary Chromatography, San Diego, CA, USA, May 1-5, 2011.
- S. K. Kim et al., “Microfabricated Gas Chromatograph for On-Site Determination of Trichloroethylene in Indoor Air Arising from Vapor Intrusion. 1. Field Evaluation”, Environmental Science & Technology 46 (11), 6065-6072 (2012).
- A. C. Lewis et al., “Microfabricated planar glass gas chromatography with photoionization detection”, Journal of Chromatography A, 1217, 768-774 (2010).
- N. E. Watson et al., “Comprehensive Three-Dimensional Gas Chromatography with Parallel Factor Analysis", Analytical Chemistry 79 (21), 8270-8280 (2007).
- E. Davydova, P. J. Schoenmakers and G. Vivó-Truyols, “Study on the performance of different types of three-dimensional chromatographic systems”, Journal of Chromatography A, 1271, 137-143 (2013).
- K. K. Unger and A. I. Liapis, “Adsorbents and columns in analytical high-performance liquid chromatography: A perspective with regard to development and understanding”, Journal of Separation Science, 35, 1201–1212 (2012). doi: 10.1002/jssc.201200042
Emily Hilder is Professor and ARC Future Fellow in the Australian Centre for Research on Separation Science (ACROSS) and School of Chemistry at the University of Tasmania. Her research focuses on the design and application of new polymeric materials, in particular polymer monoliths, in all areas of separation science. She is also interested in the development of miniaturised analytical systems, particularly for applications in clinical diagnostics and remote monitoring. She has over 95 peer-reviewed publications and was recently recognised as the LCGC Emerging Leader in Chromatography (2012). She is also an Editor of the Journal of Separation Science.