Powering a Sustainable Future with Mass Spec
How IC HRAM MS/MS can help us understand – and eventually forestall – lithium-ion battery degradation
Paul Voelker | | 5 min read | Opinion
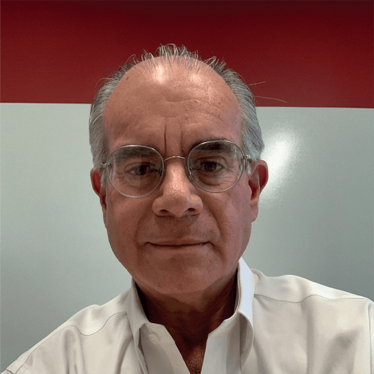
I am sure we all dearly want to move away from fossil fuels to a sustainable energy future, but there is a limitation: energy storage. Many renewable sources, such as wind and solar, to not generate a constant output of energy but, by storing energy during times of high generation, baseline demand can be met even when generation rates are low.
Similarly, when it comes to fossil-free vehicles, energy storage is again key; the energy must be portable and accessed via short charge periods and prolonged discharge over many cycles.
Lithium-ion batteries (LIBs) are the forerunners in the race to solve the full range of energy storage issues. Emerging sustainable technologies, such as next-generation electric vehicles, are already heavily invested in LIB power – an estimated 8 million LIB-powered vehicles will be on the roads by 2026 (1). Certainly, LIBs bring plenty of benefits to the sustainable storage journey; they have a high storage capacity for their size, maintain good charge/discharge cycling stability for long periods, and demonstrate minimal charge loss when not in use. But, like any storage technology, LIBs are not infallible.
Over time, battery degradation can lead to safety and performance issues that shouldn’t be underestimated. LIB issues led to Dell recalling 4.1 million computer batteries in 2006 (2) and Boeing grounding its fleet of 787 Dreamliner aircraft in 2014 (3). These problems will only magnify as we continue to shift away from fossil fuels and increase our reliance on LIBs.
As LIB use increases, so too does the dependency on rare and finite sources of lithium and the issues surrounding its extraction. Mining this rare metal is linked to geopolitical tension and environmental issues, including water diversion, biodiversity loss, and waste (4). Safe and effective recycling of the rare metal components of LIBs will be important as the industry grows, but this is also limited by the formation of degradation products.
The first step in creating safer, longer-lasting, higher-performing, and more easily reusable and recyclable LIBs is to identify degradation products, as such knowledge will help us understand the mechanistic pathways of degradation and how these can be inhibited. Here, advances in analytical technology are making a big difference. Traditional electrochemical methods have been used to measure capacity loss over time, but recent advances in ion chromatography coupled to high-resolution accurate mass (IC HRAM) MS/MS technology have provided a novel way of accurately identifying the exact ionized and ionizable components of LIB degradation.
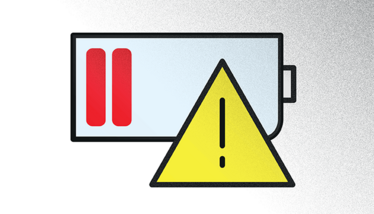
Degradation risk in every element
At a fundamental level, a LIB cell consists of a carbonaceous anode, a lithium transition metal oxide or phosphate cathode, and an electrolyte-soaked separator (the electrolyte containing a conducting salt and a non-aqueous solvent). The separator forms a permeable membrane; a barrier between the cathode and anode that prevents short circuits, but allows lithium ions to shuttle back and forth during charge or discharge. The formation of a solid electrolyte interface (SEI) on the anode helps to ensure efficient and reversible operation, enabling the LIB to keep functioning after many cycles.
This process of charge and discharge is extremely efficient and effective, but several external factors, such as elevated temperatures, charge effects, and trace contaminants, can lead to degradation. Subsequent reactions, including chemical reduction or oxidation of the electrolyte, thermal decomposition by the anode or cathode, and internal short circuits, can create degradation products. In turn, these products can reduce energy density, decrease cell life and cause safety issues, such as spontaneous ignition or explosion.
Recent studies have used IC HRAM MS/MS to identify a range of phosphate-containing electrolyte degradation products on LIB anodes. These products are of particular interest because they support several of the current degradation pathway hypotheses.
The combination of technology in IC HRAM MS/MS systems shows considerable benefit in the identification and quantification of these compounds. The IC separates the analytes based on their ion-exchange selectivity and valency, which provides valuable information on ionizable functional groups and their differentiation, even for those compounds with the same or similar unit mass. The HRAM MS/MS component provides accurate identification based on mass. Even ions, such as sulfate (SO42-) and dihydrogen phosphate (H2PO4-), both with a unit mass of 97.0, can be differentiated – showing values of 96.9601 and 96.9696 respectively with accurate mass measurements (5).
In a recent study analyzing anodes at varying stages of capacity loss, a range of compound classes and specific compounds were identified using IC HRAM MS/MS, including:
- Electrolyte breakdown products – fluoride, phosphate, and pyrophosphate.
- Ionic acids (formed from ionic compound reactions) – including phosphate esters, fluorophosphate esters, and sulfate esters.
- Organic acids (formed from the degradation of the anode).
- Methyl carbonate.
- Anionic contaminants (such as chloride and sulfate).
Already, the phosphorus-containing species identified in these studies (see Figure 1 and Table 1) support various degradation mechanism hypotheses, such as Vortmann’s pathway, based on the hydrolysis of lithium hexafluorophosphate followed by esterification of the hydrolysis and degradation products (7). Without advanced separation and identification techniques, this level of species identification was not possible.
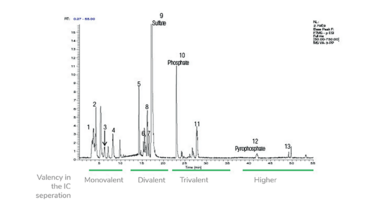
Figure 1. Full scan chromatogram of an anode wash sample from a LIB with a 45 percent capacity loss. Many of the analytes shown in the graph increased in concentration as anode capacity loss increased. Anions eluted in order of valency on the anion exchange column, but were detected as singly charged ions in the HRAM MS/MS. The inorganic ions sulphate (-2 charge), phosphate (-3 charge) and pyrophosphate (-4 charge) are noted on the graph.
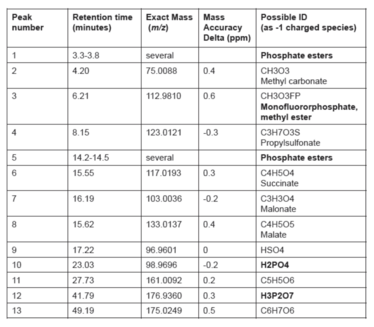
Table 1. HRAM MS/MS peak identifications for some of the species shown in Figure 1.
Powering a sustainable energy future
With the increased accuracy, separation efficiency, and detection capabilities of IC HRAM MS/MS systems, analytical scientists can now identify previously undetectable degradation products in LIBs. By identifying these compounds, analysts can explore existing theories of degradation pathways and perhaps even discover new mechanistic pathways. In turn, these findings will direct researchers to find new ways to inhibit these pathways and develop LIBs with greater safety and efficiency.
As we move closer to a more sustainable energy future supported by safe and efficient storage, understanding degradation pathways will be critical to creating LIBs with longer life and better performance. Moreover, by identifying degradation products, we can improve battery recycling and reuse, making better use of the scarce lithium resources powering this energy revolution.
- Frost and Sullivan, “Global Li-ion Battery Materials Market, Forecast to 2026” (2020). Available at: https://bit.ly/3N8EGUV
- Wired, “Dell Recalls 4 Million Batteries” (2006). Available at: https://bit.ly/3N5P4wI
- Scientific American, “How Lithium Ion Batteries Grounded the Dreamliner” (2014). Available at: https://bit.ly/3M7vpMT
- The Guardian, “The rush to ‘go electric’ comes with a hidden cost: destructive lithium mining” (2021). Available at: https://bit.ly/3M8G3TH
- Thermo Fisher Scientific, “Comprehensive Analysis of Lithium Ion Battery Anode Samples by Ion Chromatography Coupled with High Resolution Mass Spectrometry” (2016). Available at: https://bit.ly/3PicLDS
- Thermo Fisher Scientific, “Identifi cation of Phosphorus-Containing Degradation Products Obtained from Surface Deposits on Li-Ion Battery Anodes Using Ion Chromatography Coupled to High Resolution Accurate Mass MS/MS” (2016). Available at: https://bit.ly/3PfFRDQ
- B Vortmann et al., “Rapid characterization of lithium ion battery electrolytes and thermal aging products by low temperature plasma ambient ionization high-resolution mass spectrometry,” Anal Chem, 85, 6, 3433–3438 (2013). DOI: 10.1021/ac4001404
Paul Voelker is Product Marketing Manager, IC/SP DIA, Thermo Fisher Scientific