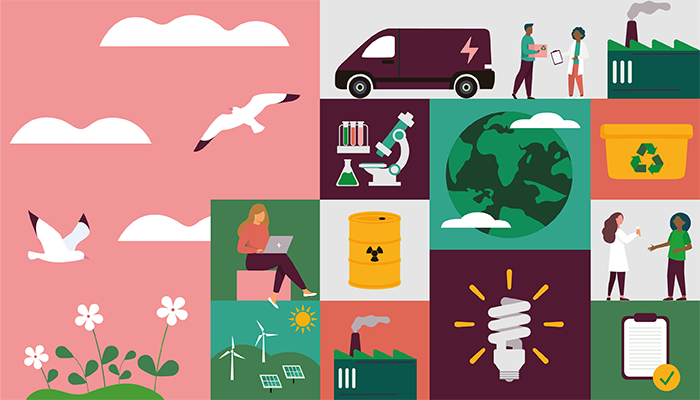
Analytical chemistry currently follows a “linear model,” where raw materials are extracted from nature, turned into products, and after use discarded as waste. Only a small fraction of used materials is cycled back into our system – placing a significant burden on the environment. We would need an endless supply of natural resources for this to work indefinitely. But the reality is we are already using way more than our planet can sustainably provide. If we keep current consumption trends, it is projected that by 2050, we will need almost three Earths to meet global demand (1).
Decoupling economic growth from resource consumption is a global priority, and circularity is seen as the key to achieving truly sustainable development. Analytical chemistry cannot afford to sit back. To stay competitive and align with global environmental goals, we must adopt circular strategies.
Circular analytical chemistry, inspired by the principles of circular economy, focuses on conserving resources, cutting waste, and protecting both the planet and human health by keeping materials in use for as long as possible (2). It uses 12 interconnected goals to guide the transition toward a closed-loop, resource-efficient, and waste-free analytical chemistry sector. It goes beyond “greening” analytical chemistry and imagines a radical transformation of the entire life cycle of products – from production to consumption to disposal. But for this to work, everyone – academia, industry, governments, and organizations – needs to work together.
The pathway to change
The 12 goals of circular analytical chemistry are as follows:
- Goal 1 aims to close material loops by promoting the collection and reuse of waste.
- Goal 2 focuses on (re)designing products and processes to prevent waste and pollution.
- Goal 3 aims to enhance resource efficiency throughout products’ life cycles.
- Goal 4 promotes adopting energy-saving technologies and transitioning to renewable energy sources.
- Goal 5 seeks to reduce the use of hazardous chemicals and processes throughout product life cycles.
- Goal 6 promotes the application of the “R-ladder,” ranking strategies like reduce, reuse, and recycle.
- Goal 7 aims to develop and apply comprehensive sustainability metrics.
- Goal 8 focuses on adopting innovative circular business models.
- Goal 9 promotes innovation through collaboration among academia, industry, and government.
- Goal 10 advocates for implementing and harmonizing policies that incentivize circular practices.
- Goal 11 emphasizes breaking free from path dependencies and lock-ins by promoting exnovation.
- Goal 12 calls for radically transforming consumption patterns in analytical chemistry.
Collectively, these 12 goals aim to facilitate a shift from the linear “take-make-use-dispose” model by creating systems where resources are used thoughtfully and sustainably. The diversity and interconnectedness of the goals allow stakeholders to prioritize those that align best with their objectives and capacities. By embracing innovative strategies that decouple product performance from resource consumption, circular analytical chemistry has the potential to revolutionize traditional workflows and position analytical chemistry as a player in the global movement toward sustainability and environmental resilience.
To put circularity into practice, we can follow four key steps that also highlight the core principles of this approach.
Step 1: Close the Loop
Closing the loop is a critical first step in achieving circularity. This can be done either by direct reuse of products and materials, by part-reuse measures such as refurbishment or – as a last resort – traditional recycling. This is certainly easier said than done, and two key factors must be addressed to get it right: “designing-out” waste and pollution and collaboration between partners.
In a well-designed circular system, efforts should be made to avoid the recycling stage at all costs. A key strategy to achieve this is by focusing on waste prevention right from the design stage. Waste often comes from poor design decisions where products, materials, and processes focus more on function and performance than on what happens at the end of their life. To fix this, we need to rethink our priorities and innovate to design-out waste and pollution. True circular products maximize resource efficiency, can be easily reused, repaired, or refurbished to extend their useful life. They can then be recycled or disassembled to create new products, with waste minimized at all stages of the extraction-production-consumption cycle.
There have been significant advancements in analytical chemistry aimed at designing-out waste, reducing environmental impacts, and improving efficiency – including several mature technologies. Solventless sample preparation based on solid-phase microextraction technologies (like solid phase microextraction (SPME) fiber, and the high-capacity versions SPME arrow, Twisters, Hisorb and thin film microextraction (TFME)) are successful examples. These technologies are solventless and reusable, while also yielding superior analytical performance compared to other traditional and hazardous sample preparation methods.
Supercritical fluid chromatography, which uses carbon dioxide as a mobile phase, is a standout innovation in reducing solvent consumption and disposal needs. The shift towards smaller particle size columns in high-performance liquid chromatography and ultra-performance liquid chromatography has minimized solvent usage while achieving the same separation quality. UPLC, in particular, utilizes smaller columns and higher pressures, offering a more solvent-efficient alternative to conventional methods, thereby cutting down both waste and resource consumption.
Although often overlooked, digital technologies like electronic lab notebooks and automated data management systems play a key role in eliminating paper waste and streamlining lab operations. By eliminating the need for paper-based records and improving data organization, these innovations help conserve resources and enhance overall lab efficiency.
Closing the loop also requires building close, mutually beneficial collaborations with all partners along the value chain, as it is unlikely that a single stakeholder can realize all activities (such as production, take-back, disassembly, recycle) alone. This is not easy – stakeholders in the analytical chemistry loop are not used to interacting and have instead focused on direct supplier and user relationships. But this will need to change.
Step 2: Improve the Loop
This step ensures that the circular approach actively addresses environmental issues and minimizes hazards by slowing resource consumption and eliminating hazardous materials. During this step, sustainability metrics can provide information on the environmental, health and safety impact of products throughout their life cycle, helping to pinpoint potential opportunities for innovation. Moreover, the “10R-ladder,” which ranks R-strategies like reduce, reuse, and recycle, is an effective tool for identifying the most impactful solutions and optimizing resource flows. To improve the loop, each stakeholder needs to dive deep into their own – as well as their partners’ – processes and activities to understand the ecotoxicological footprint along the entire loop. This includes evaluating the hazards involved in the whole life cycle of products.
Step 3: Leverage the Loop
The advantages of circularity are more widely recognized when they translate into economic profitability. After having closed and improved the loop, the next pressing question is how to maximize its value. Circular business models represent fundamentally different ways of producing and consuming goods and services, and have the potential to drive the transition toward a more resource-efficient sector. One such popular model is the sharing model, frequently implemented at an institutional level, where underutilized or expensive instrumentation is shared among researchers. Other approaches include for manufacturers to shift from simply selling analytical chemistry products to offering specific functions or services instead; there’s also the more recent chemical leasing model, which shifts suppliers’ compensation from the basis of volumes of chemicals to services delivered. To make the loop profitable, government and business policies need to be in place that support circularity goals. These should encourage the development of circular products, set standards to facilitate trade, prevent linear practices from slowing down circularity, and create the right policies to speed up innovation and overcome system lock-ins.
Step 4: Excite the Loop
Exciting the loop in analytical chemistry is all about taking action to spread and push forward circular practices. This includes getting academia, industry, and governments to work together, while also replacing old, outdated official standard methods through exnovation (the process of terminating a practice, or the use of a technology or product). We need to move beyond traditional methods that hold back innovation by supporting research into new, more sustainable materials, techniques, and processes. Educating and inspiring change is key to transforming consumption patterns in labs, which currently favor short-term product use. By raising awareness and highlighting the benefits of circular analytical chemistry, we can inspire analysts to make more sustainable, circular choices in their daily work.
According to Zero Waste Europe, single-use glass has the highest overall environmental footprint compared to other single-use materials such as plastic. However, when glass is reused at least eight times, its environmental impact is significantly reduced, becoming lower than that of plastic.
Institutions involved in biological, medical or agricultural research produce about 5.5 million tons of lab plastic waste per year, equal to around 2 percent of global plastic waste. Recycling plastics for analytical lab use can introduce additional environmental costs due to the high-quality requirements. Identifying the most frequently used plastic items, reducing their use or decontaminating/washing for reuse can lower running costs and significantly reduce the carbon footprint.
A recent analysis of the biodiversity footprint of the University of Oxford estimates that supplies and supply chains of lab equipment have much greater impacts on biodiversity overall than do international flights, the university’s consumption of electricity or its use of construction materials.
The U.S. EPA estimates that a 30 percent reduction in energy use by laboratories would decrease annual energy consumption by 84 trillion Btu. This is equivalent to the energy consumed by 840,000 households. An improvement of this magnitude would save $1.25 billion annually and reduce carbon dioxide emissions by 19 million tons – equivalent to removing 1.3 million cars from U.S. highways or preventing the harvest of 56 million trees.
The 12 goals in practice
Analytical labs already use green analytical chemistry and green sample preparation to help reduce the environmental impact of analytical work. But these concepts are typically limited to the laboratory level and tend to follow a linear approach rather than a circular one. Circular analytical chemistry goes beyond being just a set of rules to make a lab greener – it is a mindset that guides and coordinates how everyone in the sector should think, make decisions, and approach challenges, to achieve a sustainable future for all.
In circular analytical chemistry, the first and most obvious action for labs is to close the loop (Goal 1) by collecting and recycling solvents, single-use plastics, and other consumables, thus reducing waste generation. At the same time, efforts should concentrate on slowing down resource inputs and optimizing their efficiency (Goal 3). Implementing the R-ladder of Goal 6 can help hierarchize practices like reusing vials, replacing single-use laboratory consumables, repurposing materials, and recycling lab equipment.
Waste elimination is a core principle in circular analytical chemistry and this target is set through different actions rather than a unique goal. The most efficient way to eliminate waste is not to create it in the first place. This calls for academic teams to embrace innovation (Goal 9), and design new products that decouple superior analytical performance from resource consumption and eliminate pollution and waste (Goal 2). The target of waste elimination can also be reached by scaling down the number of sample analyses to just what is necessary, using chemometrics to get the needed info, or starting with simple diagnostic tools to screen samples. These steps not only cut down on waste and save resources but also reduce costs and energy consumption (Goal 4). Another effective move to save energy and resource consumption is switching to circular business models (Goal 8); for example, sharing or renting equipment instead of permanently owing it.
Analysts are familiar with the goal of minimizing hazards. Circular analytical chemistry looks at this holistically through Goal 5 and targets the whole life cycle of products from production to disposal. An example is the case of ionic liquids, deemed safe for analysts to use in the lab but often synthesized using hazardous solvents and organic reagents; and once disposed of as waste, they pose risks related to aquatic toxicity and biodegradability.
Focusing on new products and materials that are resource-efficient, prevent waste and can be cycled back may help cut down environmental, health and safety impacts. But if we don't work on changing how we consume (Goal 12), these gains are unlikely to induce long-lasting changes at a sustainable level. Increasing awareness, embracing eco-friendly laboratory "lifestyles," and promoting the benefits of circular analytical chemistry are effective ways to shift analysts' behavior towards more circular and sustainable choices – even in daily routines. Simple things like maintaining an up-to-date chemical inventory or being careful with ordering chemicals can improve lab efficiency and sustainability. Also, practices like ordering or making solutions only when needed and extending the expiry dates of homemade solutions within their stability range have been shown to reduce chemical waste by around 23 percent.
Circular analytical chemistry is about collaborating with all partners and analysts have their share in addressing the existing coordination gaps in the field (Goal 10). This is a major barrier to any circular transition because processes such as recycling demand higher levels of cooperation compared to linear chains. Laboratories can further support circularity by encouraging others in the value chain to adopt circular practices – for instance, by selecting suppliers based on environmental criteria.
The circle of life
Unlike the often vague concept of sustainability, circular analytical chemistry gives us a clear and efficient way to restore and restructure our system for long-term environmental and economic resilience. To get things right, circularity is not new – it's how nature has always worked. Natural cycles, like those of carbon, water, and nutrients, have always been circular – keeping everything balanced without generating "waste." However, this balance was disrupted with the emergence of linear “modern” civilizations and systems. The lack of value placed on natural systems, the materials extracted from them, and the harmful impacts of extractive processes have become major drivers of environmental degradation. And the waste produced within our linear approach is a major expression of this.
To tackle urgent planetary challenges such as resource depletion and climate change, we must fundamentally rethink how we make, use and dispose of analytical chemistry products. Circular analytical chemistry stands out as the most effective and forward-thinking approach to driving this transformation.
References
UN, “Goal 12: Ensure sustainable consumption and production patterns.” Available at: https://bit.ly/3FhvK0M.
- E. Psillakis, F. Pena-Pereira, “The twelve goals of circular analytical chemistry”, Trends in Analytical Chemistry, 175, 117686 (2024). DOI:10.1016/j.trac.2024.117686.