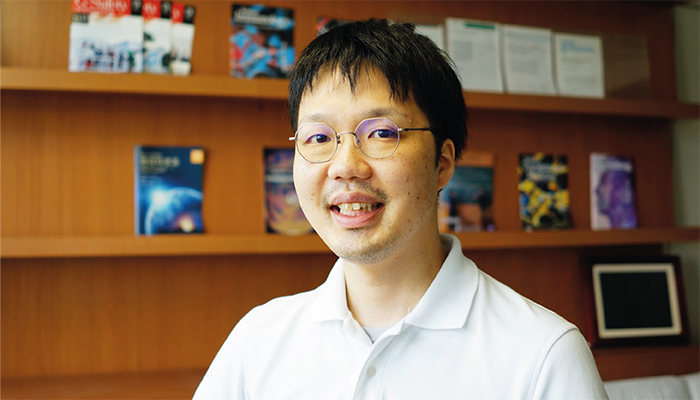
Akizumi Ishida
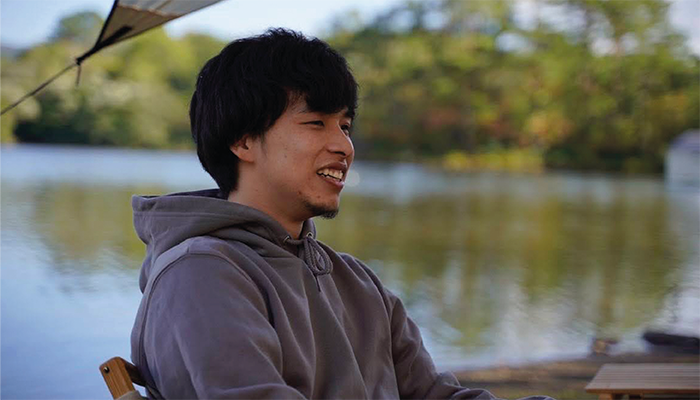
Kohei Sasaki
Last week, we reported on a new imaging technique that has allowed researchers to detect key bioessential elements, such as phosphorus and molybdenum, in 1.9-billion-year-old microfossils from the Gunflint Formation in Canada. By combining optical microscopy, electron microscopy, and high-resolution secondary ion mass spectrometry (SIMS) on a specially coated glass slide, scientists were able to obtain clear images of ancient microbial life, revealing important metabolic clues. The findings shed new light on early life on Earth.
To find out more, we reached out to co-authors of the paper, Akizumi Ishida, Assistant Professor, Department of Earth Science, Graduate school of Science, Tohoku University, and Kohei Sasaki, Researcher, Atmosphere and Ocean Research Institute, The University of Tokyo, Japan.
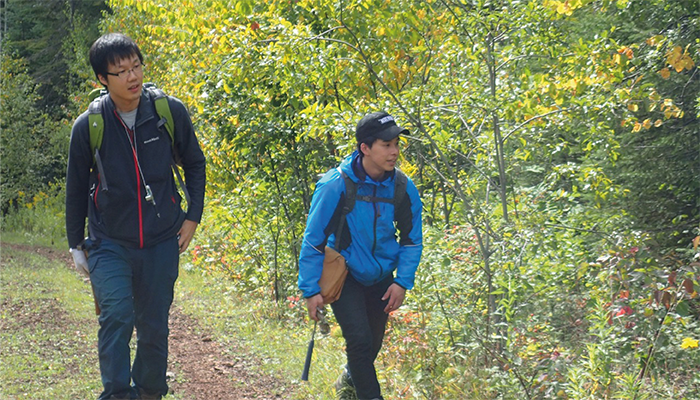
What was the main inspiration behind your new ITO-glass technique?
Ishida: The inspiration for this research came from seeing ITO glass being used when I assisted in my senior colleague's research. I was searching for a material that could retain the optical transparency needed for detailed structural observations of microfossils, while also providing conductivity suitable for electron microscopy and ion analysis. In previous studies, we analyzed microfossils by polishing rock samples, exposing the microfossils on the polished surface. However, this approach had its challenges: the microfossils we wanted to observe were often tilted relative to the polished surface, and targeting microfossils only a few microns in size during the polishing process was quite difficult. As my research progressed, I succeeded in separating the microfossils from the rock using acid treatment. Initially, I tried analyzing them on several substrate materials, such as regular glass plates and stainless steel, but none of them worked well. During this time, while assisting my senior colleague in a study on synthetic organic materials using MALDI-TOF-MS (matrix-assisted laser desorption ionization–time-of-flight mass spectrometry), which is mainly used in medical, biological, and pharmaceutical fields, I discovered that there is a type of glass (ITO glass) with a conductive coating that retains transparency. At that moment, I thought, "This is it!"
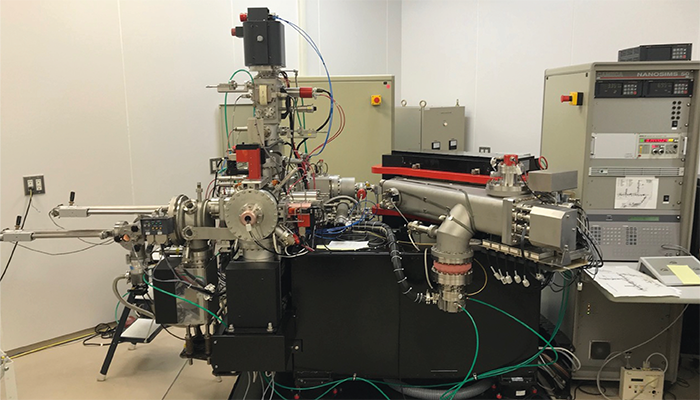
Were there any major challenges during development that you overcame? Any big eureka moments?
Ishida: One of the major Eureka moments in our research was the improved ionization efficiency of samples using our method. Our initial goal in placing "exposed" microfossils on ITO glass was to enable consistent analysis of microfossils through optical microscopy, electron microscopy, and ion imaging. However, we unexpectedly found that the ion intensity during ion analysis increased by a factor of 10 to several tens compared to when we were analyzing cross-sections of microfossils embedded in rock. We realized this was due to two factors: first, the removal of the surrounding minerals allowed the ion beam's energy to be used more efficiently for ionizing the microfossil components; second, the conductivity of the ITO glass helped to eliminate unwanted electric charge build-up during ionization. Although this was an unexpected effect, it gave us the insight that we might be able to detect not only major elements such as carbon, nitrogen, and sulfur but also trace elements that had previously been undetectable.
The detection of phosphorus from microfossils has been a long-standing challenge in microfossil research. Even in studies that have claimed success, contamination from the substrate remained an issue, and no research had definitively detected phosphorus from microfossils. In modern organisms, the phosphorus content is about 1/100th that of carbon, but for 1.9 billion-year-old microfossils, we estimated it to be around 1/10,000th to 1/100,000th. To detect this low concentration of phosphorus, we initially used a strong ion beam. As a result, we were only able to produce low-resolution imaging, barely enough to discern the shape of the microfossils. Nevertheless, when we first detected phosphorus directly from the microfossils, Dr. Sasaki, who was still a student at the time, and I jumped for joy. Afterward, Associate Professor Takahata and Professor Sano from our research team suggested ideas for improving the technique to achieve ultrahigh-resolution ion imaging by extending the analysis time while maintaining sensitivity. They also generously provided machine time for us, and we continued to make gradual improvements. In reality, it took more than five years to complete the ultrahigh-resolution imaging technique, with significant delays due to research restrictions caused by the COVID-19 pandemic.
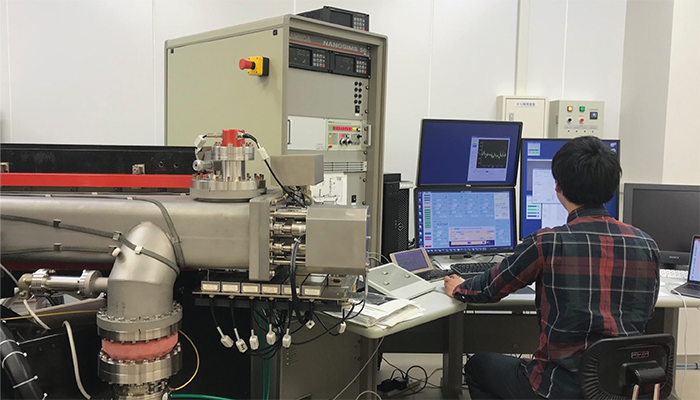
Sasaki's: One of the major challenges during the process of improving ultrahigh resolution was the presence of interfering ions with the same mass number as the target ion. For instance, phosphorus has a mass number of 31, but silicon hydride (SiH) ions also have the same mass. By increasing the mass resolution power of the instrument, we were able to clearly separate the peaks of these two ions. However, even a slight misplacement of the phosphorus peak would overlap with the tail of the SiH peak, leading to a misidentification of phosphorus. To avoid such misidentifications, we carefully adjusted the peak positions in every analysis and meticulously eliminated any potential influence from adjacent peaks. Another challenging task was the detection of molybdenum, which was one of our major discoveries. Molybdenum is a positive ion in ion analysis, which means it cannot be imaged simultaneously with negative ions such as carbon, nitrogen, and sulfur. Therefore, we attempted to detect molybdenum in the form of molybdenum oxide (MoO), a negative ion. We carefully selected molybdenum-bearing mineral, wulfenite, as a suitable standard sample for MoO ions, and we confirmed the peak positions by considering the isotopic ratios of molybdenum, which exists in multiple isotopes. Eventually, we succeeded in directly detecting molybdenum from the microfossils. Since these elements are present at low concentrations in microfossils, one frame of imaging would show almost nothing. However, by accumulating several hours' worth of frames, we were finally able to clearly see the image. The moment I first saw this, I was absolutely amazed.
One of the crucial processes in this study was the isolation of microfossils, approximately 10 µm in size, without causing any damage. Without preserving their delicate structure, we would lose the greatest advantage of these microfossils. Thanks to Professor Kakegawa of our research team, I was introduced to Professor Sugitani (Nagoya University), a professional in microfossil research, and I was able to learn the acid maceration technique he employs. This greatly advanced our research. On the other hand, residual minerals and the formation of secondary minerals after acid treatment can also lead to contamination for ion analysis. The acid separation of microfossils is a delicate and time-consuming task. We repeated the acid extraction process, which takes about a month, several times to find the optimal conditions for our samples. After successfully separating the microfossils through trial and error, we observed that not only the three-dimensional structure but even the hollow shape of the filaments was preserved. Moreover, no secondary minerals, such as fluorides, were detected on the surface, and we felt that our hard work had genuinely paid off.
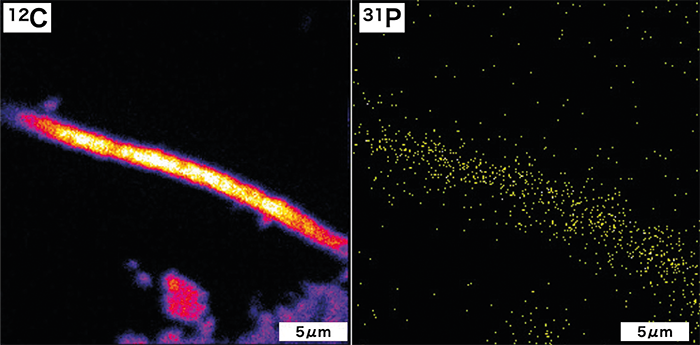
How might the techniques used here be applied to analyze other ancient samples or geological formations?
Ishida: In previous microfossil research, the major organic elements, such as carbon, nitrogen, and sulfur, have primarily been analyzed. However, our method has shown that trace elements like phosphorus and molybdenum can be analyzed while preserving the shape of the microfossils. Simultaneously, the detection of these elements in 1.9 billion-year-old microfossils provides the first clear evidence from geological samples that life, by this time, utilized phosphorus in cell membranes similar to modern life forms. Moreover, it demonstrates the potential to detect traces of enzymes that are crucial for identifying biological species. This indicates the possibility of substantiating the evolution of two of the three fundamental definitions of life: "cell membranes and metabolism" from geological samples.
Our method is applicable to microfossils from other geological eras as well. By analyzing microfossils from the Archaean eon, we can address questions about the evolution of cell membranes, such as "When did life begin using phosphorus in membrane lipids?" and "How did the enzymes responsible for nitrogen fixation evolve?" We are confident that this will provide concrete and new tools for research on the evolution of life. Conversely, by applying this method to new geological periods, we may obtain important clues regarding significant evolutionary transitions, such as the evolution from prokaryotes to eukaryotes, the emergence of larger organisms, and the evolution of multicellular life that ultimately led to modern forms.
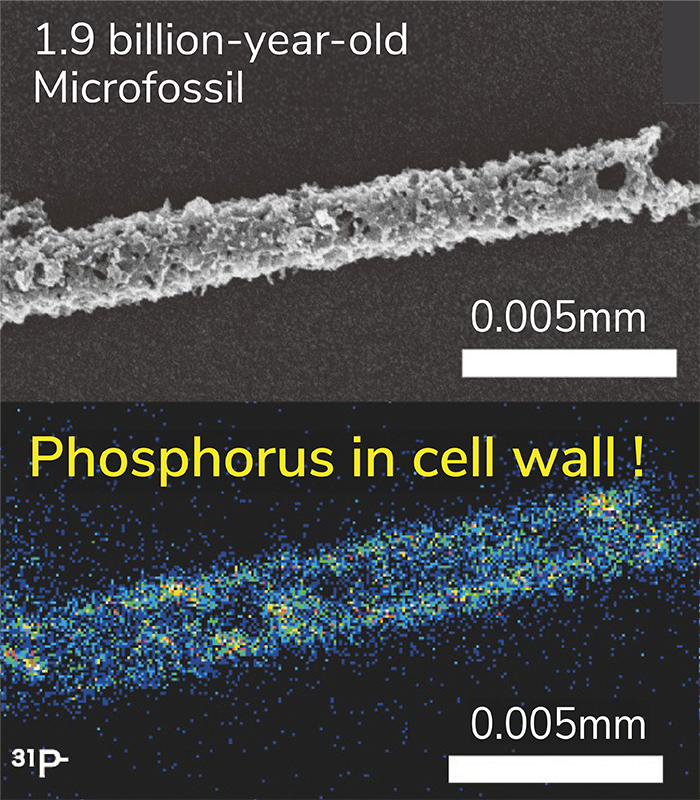
Sasaki: The era of 1.9 billion years ago serves as a milestone in the evolution of life, acting as both a starting point for exploring the evolution of early life and a foundation for tracing the evolution of modern life. In the Gunflint Formation, our research targets filamentous microfossils, but this layer also contains a diverse array of microfossil forms (referred to as Gunflint microfossils). For instance, microfossils with internal structures resembling nuclei have been discovered. By clarifying the elemental distribution in these microfossils, we may uncover clues regarding the evolution from prokaryotes to eukaryotes.
While this paper focused on molybdenum, there are trace elements in enzymes and chemical substances, such as iron and vanadium in nitrogenase (nitrogen-fixing enzymes) and magnesium and zinc in chlorophyll, that can also differentiate between types of microbes. In the future, we aim to elucidate the differences in membrane structures and traces of enzymes corresponding to the morphology of Gunflint microfossils, achieving results that could serve as “standard samples” in microfossil research. Furthermore, as we accumulate such knowledge, our methods could be applied to the analysis of amorphous organic materials, such as shape-less biofilms, kerogen, and petroleum. We hope to expand our research focus to address broader issues, such as modern analog studies using contemporary microbial biofilms and the supply of prebiotic organic matter to Earth from amorphous organic materials found in meteorites.
Images credit: Supplied by Interviewee