Shifting the Landscape of Nanomaterial Measurement
Collaboration between the University of Montreal and PerkinElmer shows how the unique capability of single particle inductively coupled plasma-mass spectrometry (SP-ICP-MS) can be used to assess the environmental impact of engineered nanomaterials.
Chady Stephan, Madjid Hadioui, Kevin J. Wilkinson, Robert Thomas |
sponsored by Perkin Elmer
The Problem
The recent surge in the use of engineered nanomaterials (ENMs) in consumer products has resulted in concerns about their release into the environment. To properly assess their impact on ecological and human health, it is necessary not only to predict exposure through modeling, but also to perform quantitative physical and chemical measurements. Historically, metrics like particle size have been carried out using techniques such as dynamic light scattering (DLS), nanoparticle tracking analysis (NTA) and transmission electron microscopy (TEM), while dissolved content has been typically measured by ultrafiltration followed by inductively coupled plasma mass spectrometry (ICP-MS). However, these traditional techniques have known limitations when it comes to quantifying very low levels of ENMs in the presence of natural colloidal species in complex waters. As a result, they are not ideally-suited for real-world environmental matrices, where nanoparticle concentrations are typically extremely low.
The Solution
Single particle inductively coupled plasma mass spectrometry (SP-ICP-MS) is an exciting new technique for detecting and characterizing metal nanoparticles (NP) at very low concentrations. SP-ICP-MS is fast and can provide significantly more information than other traditional techniques, including particle number concentration, particle size, and size distribution, in addition to the concentration of dissolved metals in solution. The added benefit of using SP-ICP-MS is that it can distinguish between particles of different elemental compositions.
SP-ICP-MS is based on the measurement of the signal intensity produced by a single particle. Nanoparticle suspensions are sufficiently diluted to make sure that only a single particle reaches the plasma at a time, where it is atomized and ionized, producing a signal of relatively high intensity that is measured as a pulse. Figure 1 shows particles (P) and dissolved analyte in the sample aerosol entering the plasma and being ionized. As the ions pass through the interface region into the ion optics where they are eventually separated in the mass spectrometer, particles are detected as individual pulses, whereas the dissolved analyte contributes to a continuous shift in the background signal. The frequency of pulses (events) provides the particle number concentration, whereas the intensity of each pulse is proportional to the mass of the nanoparticle. Because of the short transient nature of the pulse, very short integration times are necessary to maximize the detection of individual particles as pulses of ions after they are ionized by the plasma.

Figure 1: A suspension of nanoparticles (P) and dissolved analyte reaches the plasma where each particle is ionized, producing a signal that is measured as a single pulse.
Figure 2 shows both metal nanoparticles and metal ions in solution being ionized by an ICP-MS system (NexION 350X, PerkinElmer Inc., Shelton, CT). The signal from the dissolved ions is represented by the continuous signal below the dashed line, while the ionized pulses of nanoparticles are represented by the individual spikes.
For this approach to work effectively, the speed of data acquisition and the response time of the detector must be fast enough to capture the time-resolved NP pulses, which are typically 300-400 µs (1). If the electronics are not fast enough, two or three pulses can easily pass through in one single integration leading to inaccurate particle counting and sizing. For this application, the ICP-MS should be capable of using dwell times shorter than the particle transient signal time, thus avoiding false signals generated from clusters of particles. In practice, for single element nanoparticle studies, this means using a dwell time of less than 100 µs and a settling time of zero, so the pulse can be fully characterized and precisely integrated using a peak area integration algorithm (2).
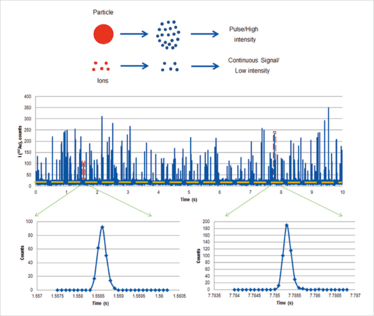
Figure 2: Metal nanoparticles (pulses) and metal ions in solution (continuous signal below the yellow-dashed line) being ionized in the plasma.
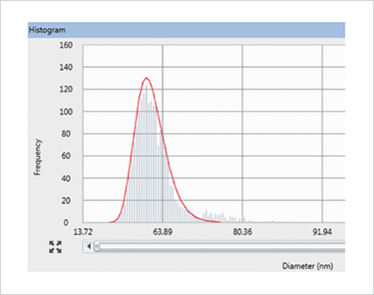
Figure 3: A size distribution histogram of 60 nm diameter gold particles showing frequency of ion pulses (events) plotted against nanoparticle diameter.
Once generated, the signal intensity as a function of time can then be processed using a theoretical approach, first developed by Duegeldre and co-workers (3). In this approach, a histogram of NP diameter versus number of events (NP frequency/number) is generated in order to characterize the NP distribution in the sample. Figure 3 shows a size distribution histogram of 60 nm gold particles, with frequency of ion pulses (events) plotted against nanoparticle diameter, generated using PerkinElmer’s Syngistix Nano Application Software Module (4).
Beyond the Solution
SP-ICP-MS is a very exciting development for the identification and characterization of nanoparticles at environmentally-significant levels and is being used to improve our understanding of how nanomaterials are interacting with the outside world. However, the technique is continually being fine-tuned to improve its detection capability even further. One of the main difficulties in the data processing for SP-ICP-MS is the discrimination between the intensities of dissolved metal and ENP. This is particularly difficult for high concentrations of dissolved metal and/or small ENP sizes. With no silver present in solution, the minimum detectable size is 11 nm, whereas a concentration of 2 µg/L of Ag+ would degrade the detection capability approximately five-fold.
It is therefore extremely important to be able to remove or at least reduce this limitation, given that the smallest ENPs are most likely to be of greatest environmental risk. It should also be emphasized that particle solubility increases with decreasing particle size, so the smallest particles will always be the most difficult to quantify.
While some groups have used statistical methods or iterative software algorithms to remove the contribution of the continuous signal, recent research activity has focused on coupling SP-ICP-MS with separation techniques such as field flow fractionation (FFF) (6), or hydrodynamic chromatography (HDC) (7). One of the more promising approaches is to use ion binding columns coupled to the SP-ICP-MS to remove the dissolved metal ions in solution (5). Figure 4 shows a mixture of 0.05 µg/L of 20 nm Ag nanoparticles, 0.09 µg/L of 60 nm Ag nanoparticles spiked with 1.2 µg/L of Ag+ in solution. Figure 4a shows the raw signal for the nanoparticle and dissolved silver analyte, while Figure 4b shows the derived particle size distribution. Under conditions of high dissolved metal, it is not possible to distinguish the smaller Ag nanoparticles from the background Ag. But by using ion exchange resins, it is possible to lower background noise sufficiently so that the 20 nm Ag nanoparticles are detected. For example, when a SiSH (SiliaMetS Thiol, SiliCycle) resin, which is highly specific for Ag+, was employed, the background signal decreased substantially, as seen in Figures 4c and 4d, resulting in the easy discrimination of the smaller nanoparticles.
Somewhat surprisingly, a similar result was achieved when the sample was passed through a non-ionic, hydrophobic resin (Amberlite XAD 1180N), which is typically used to remove natural organic matter (NOM) from aqueous samples. This is shown in Figures 4e and 4f. For both resins, dissolved silver decreased, significantly reducing the background signal, whereas the resolution and identification of the particulate silver fraction improved substantially, as demonstrated in Figures 4d and 4f. In both cases, the signal intensity attributed to the nanoparticles was constant, confirming that the nanoparticles did not appear to interact with either of the resins.
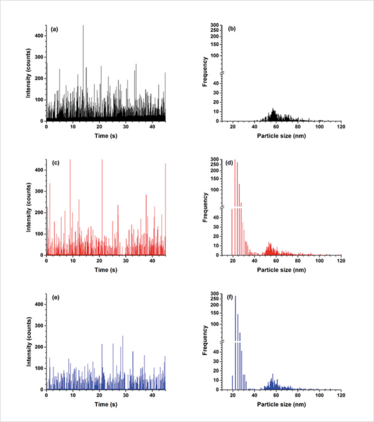
Figure 4: Signals from a sample mixture of 0.05 µg/L of 20 nm Ag nanoparticles, 0.09 µg/L of 60 nm Ag nanoparticles spiked with 1.2 µg/L of Ag+ in solution (4a), and the reduction of background signal from the dissolved silver after being passed through a SiSH resin (4c) and XAD resin (4e) demonstrating that greater discrimination of the nanoparticle pulses from the dissolved metal can be achieved. Derived size distributions are shown with no resin (4b), SiSH resin (4d), and XAD resin (4f) for the sample mixture described.
Much of the data presented in this study suggests that coupling ion exchange resins to SP-ICP-MS will further enhance the capability of SP-ICP-MS by detecting smaller-sized nanoparticles, particularly in the presence of other matrix elements. Under these conditions, where both the nanoparticles and ions were present, Hadioui and co-workers (5) have shown that this technique is capable of enhancing the sensitivity by a factor of 2-fold, resulting in a significant increase in the signal to noise ratio (5).
Summary
The excellent elemental sensitivity and specificity of ICP-MS makes it ideally-suited for the characterization of metal-based engineered nanomaterials at environmentally-relevant concentrations. In particular, using the technique in the single particle analysis mode allows more information to be obtained in a much shorter time, such as understanding how much of the nanoparticle has dissolved in the sample and how much is still in the suspended particulate form. In addition, recent developments of coupling SP-ICP-MS with ion exchange column technology is further improving its flexibility and detection capability and allowing this approach to be used for real-world environmental samples. However, it should be emphasized that measuring nanoparticles with ICP-MS in Single Particle mode is quite different to measuring dissolved species. For this reason, it is very important that the speed of data acquisition and the measurement protocol be optimized to detect and process these rapid transient events.
Chady Stephan is Manager, Global Applications-Nanotechnology at PerkinElmer, Inc., Shelton, CT, USA; Kevin J. Wilkinson is a Professor and Madjid Hadioui is a Research Associate, both at the Université de Montréal, Canada; and Robert Thomas is Principal Consultant at Scientific Solutions, Gaithersburg, MD, USA.
- J. W. Olesik and P. J. Gray, “Considerations for Measurement of Individual Nanoparticles or Microparticles by ICP-MS: Determination of the Number of Particles and the Analyte Mass in Each Particle”, J. Anal. At. Spectrom., 27, 1143, (2012).
- A. Hineman and C. Stephan, “Effect of Dwell Time on Single Particle Inductively Coupled Plasma Mass Spectrometry Data Acquisition Quality”, J. Anal. At. Spectrom., 29, 1252-1257, (2014).
- C. Degueldre and P. Y. Favarger, “Colloid Analysis by Single Particle Inductively Coupled Plasma-Mass Spectroscopy: a Feasibility Study”, Colloids and Surfaces A: Physicochem. Eng. Aspects, 217, 137–142, (2003).
- Syngistix Nano Application Module for Single Particle ICP-MS: PerkinElmer Application Study, www.perkinelmer.com/catalog/product/id/N8140309
- M. Hadioui, C. Peyrot, and K. J. Wilkinson, “Improvements to Single Particle ICPMS by the Online Coupling of Ion Exchange Resins”, Anal. Chem., 86, 4668−4674, (2014).
- D.M. Mitrano, et al., “Silver Nanoparticle Characterization Using Single Particle ICP-MS (SP-ICP-MS) and Asymmetrical Flow Field Flow Fractionation ICP-MS (AF4-ICP-MS)”, J. Anal. At. Spectrom., 27(7), 1131-1142, (2012).
- K. Proulx and K.J. Wilkinson: Separation, detection and characterization of engineered nanoparticles in natural waters using hydrodynamic chromatography and multi-method detection (light scattering, analytical ultracentrifugation and single particle ICP-MS); Environmental Chemistry, 11(4), 392-401, 2014
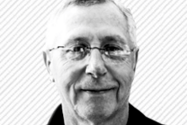
Robert (Rob) Thomas was raised in the mining and industrial communities of South Wales in the UK in the 1960s, where social activities revolved around playing rugby, drinking beer and complaining about the incessant rain! When he finally graduated from Gwent College of Technology, with a graduate degree in chemistry in 1973, he realized there was a bigger world out there and ventured out to experience the bright lights and gold paved roads of the London area, where he ended up working for a US-based analytical instrumentation company. He spent 10 years learning how to communicate with the English-speaking world and applying his chemistry knowledge to the field of Atomic Spectroscopy.
The parent company soon learned of his immense skills and transferred him to Connecticut, USA, in 1987, where he eventually ended up specializing in the field of ICP-MS. Along the way he has become a Fellow of the Royal Society of Chemistry (FRSC) and also a Chartered Chemistry (CChem). He is accredited with over 70 publications on analytical chemistry and 3 textbooks on ICP-MS. For the past 13 years he has been principle of his own consulting company, based in Gaithersburg, MD.