Stable Isotopes in an Unstable Climate
The ongoing search for evidence of our changing environment
Calum Preece |
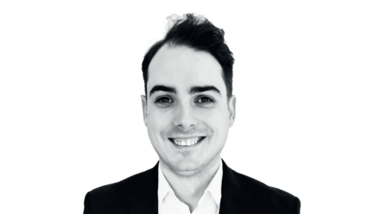
Since the 1860s, when physicist John Tyndall recognized the Earth’s natural greenhouse effect, scientists have understood the insulating qualities of carbon dioxide and other greenhouse gases. A seminal paper written by Swedish scientist Svante Arrhenius in 1896 was the first to predict that changes in the level of carbon dioxide in the atmosphere could substantially alter the surface temperature, further advancing our understanding.
Today, satellites and other technological advances have enabled scientists to collect more information about the Earth, allowing them to analyze its climate on a global scale and to identify the signs of a changing climate.
How the Earth’s climate responds to changes in greenhouse gas can be seen in ice cores drawn from Greenland, Antarctica, and tropical mountain glaciers. Bubbles of air in glacial ice trap samples of the Earth’s atmosphere from as far back as 800,000 years ago, and the chemical makeup of the ice provides clues to the average global temperature. Additionally, the study of tree rings, ocean sediments, coral reefs, and layers of sedimentary rock all allow scientists to see the Earth’s atmospheric conditions as they existed thousands of years ago.
Using these ancient records, scientists have been able to see how the Earth’s climate has changed over time, including past ice ages and periods where the climate was warmer than it is today. This paleoclimate record also reveals that current climatic warming is occurring more than 10 times faster than the average rate of ice age warming (1). The predicted rate of warming for the next century is at least 20 times faster.
But scientists studying climate change to determine future scenarios only have what has happened before to help their research. Stable isotope analysis works as a virtual paleo-thermometer, allowing readings of past Earth temperatures in a variety of materials. By combining this temperature information and extrapolating into the future, stable isotope analysis plays a crucial role in helping to understand how the worst outcomes can be avoided.
For example, Peter Wynn, a senior lecturer at Lancaster University, uses stable isotope geochemistry in glaciology and the reconstruction of paleoclimates. Wynn and his team conduct stable isotope analysis on a diverse range of sample types and across a broad range of environmental problems – from deep geology and pollution dynamics to wildfowl migration patterns (2).
The department specializes in analyzing sulfur isotopes and high-temperature oxygen pyrolysis. And they have established techniques to extract sulfur isotopic signals from paleo records, including speleothems, tree rings and carbonate rocks, and routinely use high-temperature pyrolysis to extract the isotopic record from oxygen-bearing molecules, including sulfates and phosphates. Future research for the department will focus on isotope analysis of greenhouse gases, including the analysis of methane C and H isotopes and nitrate N and O isotopic composition in a variety of environmental samples.
Another example: research engineers Michel Stievenard and Monique Pierre supervise a stable isotope laboratory of the continental paleoclimate section of the Laboratoire des Sciences du Climat et de l’Environnement (LSCE) (3). The group analyzes cellulose from tree rings for their carbon and oxygen isotopic composition – data that provides insight into past climates, especially humidity and temperature conditions. The team reconstruct regional climates of the past using trees from places all over the world; for example, living trees from Tibet and Morocco, Réunion island fossil trees, and massive wood beams from historical French castles. One project focused on finding a reliable paleoclimate reconstruction based on tree ring proxies (4).
The project compared the potential of the oxygen isotopic composition of the cellulose in two types of trees: Fitzroya cupressoides and Nothofagus pumilio, which are found in Patagonia. The F. cupressoides was favoured to assist in long-term climate reconstruction due to their longevity (they can grow up to almost 4,000 years old). However, due to a poor understanding of its complex physiology, it was difficult to determine the climatic signal recorded in its δ18Ocell. The results indicated that N. pumilio represented a more robust paleoclimate archive due to its δ18Ocell consistency between sites. Analyses of a 200-year-long δ18Ocell chronology are currently in progress.
Climate change is one of the greatest threats to human civilization, but determining future climate scenarios can only be built on the foundation of what we know has happened before. In order to achieve this, the world’s scientific community will need to make full use of all of the tools available to them - which is why elemental analysis, which has been long-established as a tried-and-tested method of long-term climate analysis, will be relied upon more than ever before.
Leading scientific organizations, such as NASA, have cited elemental analysis as one of the key tools they rely upon to extract the maximum amount of climate insight from the physical, chemical and biological materials used as climate proxies, meaning climatologists now have a strong idea of how global temperature trends have developed for millions of years into the past. Armed with this knowledge, it becomes possible to develop well-informed climate scenarios for the future, which in turn clarifies the actions that must be taken if the world is to avoid the worst possible outcomes.
As with all aspects of the current climate crisis, knowledge of these factors is only half the battle, and the world still faces many obstacles to making the necessary difference. However, by making use of the full range of technological solutions available, it will be possible to ensure that any future decisions are taken from the best possible scientific vantage point.
The Consequences of Climate Change
From the study of past climates, many factors influence changes: solar activity, oscillations of the Earth’s orbit, greenhouse gases, ice cover, the abundance or lack of vegetation on the land, the configuration of continents, dust thrown up by volcanoes or wind, the weathering of rocks, and so on. However, the details are not as clear and there are complex interactions that can amplify or dampen changes to the climate. For example, while sea ice reflects more of the sun’s energy than open water, it can trap heat in the water beneath the ice.
To understand what is causing the current rapid warming, we need to consider more than the natural variations by including the impact that human activity has had on the rise in temperature.
The Earth’s average surface temperature has risen about 0.9 ℃ since the late 19th century – a change driven largely by increased carbon dioxide and other anthropogenic emissions into the atmosphere (5). This has resulted in the following:
Warming oceans. The ocean absorbs vast quantities of heat as a result of increased concentrations of greenhouse gases in the atmosphere, mainly from fossil fuel consumption. The Fifth Assessment Report published in 2013 by the Intergovernmental Panel on Climate Change (IPCC) revealed that the ocean had absorbed more than 93 percent of the excess heat from greenhouse gas emissions since the 1970s, which is causing ocean temperatures to rise.
Shrinking ice sheets. Both the Greenland and Antarctic ice sheets have decreased in mass, according to data from NASA’s Gravity Recovery and Climate Experiment. The Greenland ice sheet has lost an average of 281 billion tonnes of ice per year between 1993 and 2016, while the Antarctic sheet has lost about 119 billion tonnes over the same time period, which indicates a threefold acceleration of the loss rate in the last decade (6).
Declining Arctic sea ice. The Arctic sea ice has seen a decline in its spatial extent and thickness over the last several decades. Typically, sea ice covers about 14 to 16 million square kilometres in the late winter in the Arctic, while the seasonal decrease is approximately 7 million square kilometres at the end of summer. Over the past 10 years, the remaining sea ice has only measured 3.5 to 5 million square kilometres (7).
Glacial retreat. Almost all the glaciers around the world, including in the Alps, Himalayas, Andes, Rockies, Alaska and Africa, are retreating, with some glaciers showing unprecedented retreat rates over that last century (8).
Reduced snow cover. Satellite observations have revealed that there has been a decrease in the amount of snow cover in the Northern Hemisphere during spring and summer, and that the snow is melting earlier in the spring. This means the ground is absorbing more of the sun’s energy, warming the planet (9).
Rise in sea level. Over the last century, the global sea level has risen by approximately 200 mm, while the rate in the last two decades has nearly doubled compared with that of the last century (6). If CO2 and other greenhouse gases continue to increase, projections suggest that the sea level may rise by a further 500–1000 mm by 2100 (10).
Extreme events. The number of extreme weather events has increased as a result of climate change. As the Earth’s lower atmosphere becomes warmer and increasingly moist due to human-emitted greenhouse gases, the potential for storms and certain extreme weather events grows (11).
Ocean acidification. Since the Industrial Revolution, the acidity of surface ocean waters has increased by about 30 percent – the result of increased levels of anthropogenic carbon dioxide being released into the atmosphere (12).
- NASA (2010). Available at: https://go.nasa.gov/3CBm4qX
- Elementar UK (2021). Available at: https://bit.ly/3CHvl0Y
- Elementar UK (2021). Available at: https://bit.ly/3oKVcjB
- M. Stievenard, et.al. (2020). Available at: https://bit.ly/3nF4fTS
- B.D. Santer et.al., “Contributions of Anthropogenic and Natural Forcing to Recent Tropopause Height Changes,” Science vol. 301 (25 July 2003), 479-483.
- JPL (2018). Available at: https://go.nasa.gov/3BGC8rQ
- NSIDC (2021). Available at: https://bit.ly/2YOBfPq
- NSIDC (2021). Available at: https://nsidc.org/cryosphere/sotc/glacier_balance.html
- NSIDC (2021). Available at: http://nsidc.org/cryosphere/sotc/snow_extent.html
- IPCC (2018). Available at: https://bit.ly/2YQ1BkE
- The Royal Society (2020). Available at: https://bit.ly/3j4pj3n
- NASEM (2016). Available at: https://bit.ly/3cyGA0U
- PMEL (2021). Available at: https://bit.ly/3FtOKUO
Calum Preece, Environment Product Manager, Elementar UK