An Elemental Regeneration
Emerging applications in life sciences and environmental analysis are driving renewed interest in the unique properties of ICP-TOFMS – in particular, its ability to detect complete elemental mass spectra from short transient events, such as single nanoparticles.
Alexander Gundlach-Graham |
Prior to moving to Switzerland, I did my PhD at Indiana University in Gary Hieftje’s group, where I studied a new form of velocity-based mass spectrometry – distance-of-flight mass spectrometry (DOFMS). My research into DOFMS was formative, and taught me about mass spectrometer design, construction, and operation. I’ve carried the interest and expertise I gained in velocity-based mass spectrometry to my current work at ETH Zurich, developing analytical methods for the high-throughput analysis of diverse inorganic nanoparticles from environmental samples by ICP-TOFMS.
As I began searching for a postdoc position, I was aware of renewed interest in full-spectrum atomic mass spectrometry, largely because of key applications in biomolecule detection and laser-ablation imaging. The potential encouraged me to join the Günther group, who were central to the revival of inductively coupled plasma time-of-flight mass spectrometry (ICP-TOFMS).
ICP-TOFMS rediscovered
ICP-TOFMS is not a new technique; it was pioneered in the early 1990s by the Hieftje group and then commercialized by two instrument companies. However, ICP-TOFMS had limited commercial success because the sensitivity and dynamic range lagged too far behind other scanning-based ICP mass spectrometers. Notably, the initial limitations of ICP-TOFMS were largely the result of the inadequacies of high-speed digitization electronics at the time. Today, high-speed detection electronics have improved (and continue to do so), so ICP-TOFMS has matured into an approach that can compete with other ICP-MS instrument designs in terms of sensitivity, limits of detection, and linear dynamic range. Moreover, it offers unique measurement possibilities through high-speed, full-spectrum ICP-MS analysis.
In my time at ETH Zurich, I’ve worked predominantly with two ICP-TOFMS instruments: a prototype instrument that was developed through a collaboration between TOFWERK (Thun, Switzerland) and the Günther group, as well as a commercial ICP-TOFMS instrument (icpTOF, TOFWERK). The collaboration between the Günther group and TOFWERK is a great example of how a university-based research group can work in symbiosis with a company in developing a new tool; the academic group provides vision and expertise in compelling and significant applications, while the company provides technical know-how and the environment to develop a user-ready instrument.
I work mainly on two applications: elemental imaging with laser ablation, and single-nanoparticle detection and quantification. In both of these research areas, ICP-TOFMS provides measurement characteristics that are not available and often fundamentally not possible on other elemental mass spectrometers. The key benefit of ICP-TOFMS is that it provides full spectral coverage and very rapid data acquisition speeds. For laser ablation and single-particle analysis this is a major advantage because it means that we can measure complete atomic mass spectra for discrete transient events, such as single laser ablation events or single nanoparticles. From a practical standpoint, full mass spectrum detection is also beneficial because it simplifies mass spectrometer experimental setup and means that we never miss any unexpected – but pertinent – isotopic signals.
New approach, new measurements
In laser-ablation ICP-MS, a pulsed laser is used to ablate a small quantity of a solid material and the resultant aerosol is transported to the ICP-MS, where the elemental and isotopic composition of the ablated aerosol is measured. Laser ablation is a clean way to sample solid materials and, by controlling the position of the laser, it can be used to measure sample material with lateral and depth resolution. Combined with ICP-MS, laser ablation can provide element and isotope composition at high lateral resolution. Laser ablation is fundamentally a pulsed technique: a laser impinges on a material surface, which causes ablation, and a cloud of sample material is generated – all in a timescale of nanoseconds to hundreds of microseconds. In conventional LA-ICP-MS, sample transport systems are used to “smear” laser-ablated aerosol particles from many laser-ablation events so that timescale of the mass spectrometry analysis matches that of the laser-ablation sampling. Spreading the time profile of LA aerosols leads to more accurate quantification for analysis with scanning-based ICP-MS instruments; however, it also means that information about each LA shot is lost and that lateral and depth resolution is limited by the overlap of signals from temporally (and physically) adjacent laser shots. The high-resolution LA-ICP-TOFMS system we’ve developed in the Günther lab is fundamentally different to this conventional LA-ICP-MS approach.
In our lab, we use a “pulse-resolved” LA-ICP-TOFMS imaging strategy – the complete transient signal of each LA event is measured without overlap from adjacent LA event signals. This imaging strategy prevents the image blur that arises when ablated aerosols from different locations are mixed in the aerosol transport system. To perform high-speed pulse-resolved LA-based imaging, research in the Günther lab has focused on development of a low-dispersion LA cell and aerosol transport system that can deliver ablated aerosol from individual laser-sampling events within 10 ms. Several other groups are also pursuing low-dispersion LA aerosol transport; however, to provide meaningful and quantitative information about the material sampled at each LA position, we need an ICP-MS instrument that has the speed to match the laser sampling – this is where ICP-TOFMS comes in. By combining low-dispersion LA sampling with ICP-TOFMS, we are able to generate multi-elemental high-dynamic-range quantitative elemental images at lateral resolutions down to five µm and at high speed (100 pixels/sec). The figures of merit of LA-ICP-TOFMS imaging are simply not available with other ICP-MS instrument designs.
More importantly, the elemental images that can be generated with this approach are compelling and offer access to otherwise unavailable information about trace-, minor-, and major-element spatial distributions across a specimen. In my research, I have focused on the application of LA-ICP-TOFMS imaging for analysis of geological samples, where high-resolution elemental imaging combines with observations about specimen textures to provide insight into rock formation and history. LA-ICP-TOFMS has also been shown to be very promising for elemental bioimaging. By staining tissues with isotope-labeled antibodies and performing LA-ICP-TOFMS imaging experiments, highly multiplexed distributions of biomolecules can be measured at subcellular lateral resolution. This approach is called imaging mass cytometry (commercialized by Fluidigm, USA) and was partly developed in collaboration with our lab. Apart from antibody-labeled elemental imaging, LA-ICP-TOFMS will also have substantial impact in the burgeoning field of elemental bioimaging, where LA-ICP-TOFMS approaches will help researchers hone their understanding of the roles and diagnostic potential of native metals in biological systems.
Addressing a (nano)particular problem
Another promising application of ICP-TOFMS is single-particle ICP-MS analysis, in which particle number concentrations of nanoparticles and elemental compositions of single-nanoparticles are measured. The expanding use of inorganic nanoparticles in a variety of consumer goods (everything from lotions to fabrics to food packaging) and industrial processes, makes measurement of individual nanoparticles, especially in complex natural media, an emerging analytical challenge. Nanoparticles are hard to detect – they are small (hundreds to millions of atoms), dilute in terms of total mass concentration, and often present in matrices that contain naturally occurring colloids with similar element compositions.
We have a good understanding of how nanoparticles behave within the products or processes for which they are intended; however, their eventual fate is harder to predict. Nanomaterials make their way into the environment through many routes, whether through disposal into water or solid-waste handling systems that are not designed to deal with nanomaterials, or through incidental release, such as from automotive exhaust. Predicting the impact of nanomaterials and nanoparticles being released into the environment requires the modeling of particle fate and transport, understanding routes of exposure, and performing toxicology experiments. All of these studies need to be supported by analytical measurements. It’s important that we better understand the amount and chemical species of inorganic nanoparticles in natural systems, and develop systems for monitoring them.
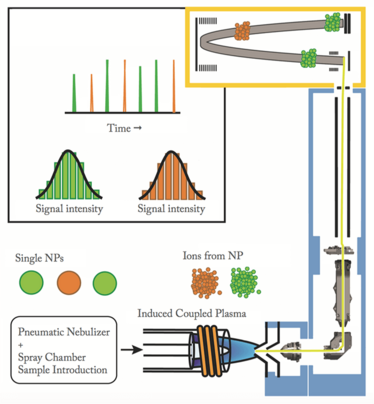
Figure 1. In single-particle ICP-TOFMS (schematic of icpTOF shown here), dispersed NPs are introduced into the ICP, where they are vaporized, atomized, and ionized. Each NP produces a dense cloud of ions that is recorded as a short burst (300–500 µs) of signal by the mass spectrometer. The magnitude of the single-particle signal correlates to particle mass and the frequency of bursts to particle number concentration. With ICP-TOFMS, ions of all m/z are measured simultaneously, so a diverse range of NPs (including multielemental NPs) can be analyzed in a single run.
Multi-Element Single-Particle ICP-TOFMS Signal
Currently, there is no single measurement (or even a defined group of measurements) that is used to characterize a broad range of nanoparticles in natural systems. We are working to develop single-particle ICP-TOFMS as a comprehensive measurement system for non-targeted analysis of diverse inorganic nanoparticles. In single-particle ICP-MS, liquid samples with dispersed inorganic nanoparticles at environmentally relevant concentrations (~105 particles/mL) are introduced into the ICP-MS instrument. When a nanoparticle passes through the ICP, it is vaporized, atomized, and ionized so that a dense cloud of ions is produced and detected as a signal burst by the mass spectrometer. If this signal burst is more intense than dissolved background signal levels, we are able to count the particles. In fact, single particle-ICP-MS can even detect nanoparticles in a matrix that has a higher mass concentration of analyte in the dissolved fraction than the nanoparticulate fraction. Because the ICP is a robust atomization and ionization source, we can also quantify the amount of element(s) in each nanoparticle. With conventional ICP-MS instruments, only a single isotope can be monitored across the short timescale of a single-particle event; TOFMS allows for all elements to be measured within each single-particle event. By using ICP-TOFMS, we can measure both multi-elemental signatures of individual particles and a diverse range of nanoparticle types – in a single analysis.
To illustrate the advantages of ICP-TOFMS for multi-element detection of single nanoparticles, consider our proof-of-principle study, measuring engineered nanoparticles of cerium dioxide (CeO2) in a soil matrix with cerium-containing natural nanoparticles. In this study (1), we were able to distinguish the two types of cerium particles (engineered and natural), based on conserved multi-element fingerprints from each nanoparticle type. With a machine-learning classification algorithm, we were able to quantitatively count engineered nanoparticles in a matrix with over 100× more natural than engineered cerium nanoparticles. Importantly, without TOFMS, we would have only been able to measure the signal of 140Ce+ for each nanoparticle, and could not have differentiated the Ce-containing natural nanoparticles from the CeO2 engineered nanoparticles – single-particle ICP-TOFMS has enabled a real leap forward in measuring inorganic nanoparticles in complex matrices.
ICP-TOFMS impact
I have described just a few of the many applications where fast, multi-element analysis is critical. Other applications primed for ICP-TOFMS include multiplexed isotope tags for biomolecule quantification, and combination with fast separations. I believe that this next generation of commercial ICP-TOFMS instruments will become a mainstay in atomic mass spectrometry. Such instruments provide sufficient sensitivity for most trace-element analysis laboratories and offer measurement capabilities – high-speed full-spectrum detection – to meet the challenges of contemporary elemental analysis. Even more exciting is the potential for more powerful ICP-TOFMS instrumentation in future; higher sensitivity and dynamic range is certainly achievable. Emerging applications – especially in biological sciences and environmental analysis – that simply cannot be tackled with alternative elemental mass spectrometry approaches will no doubt drive further development of ICP-TOFMS. All in all, it is a fun time to be working with ICP-TOFMS – and a thrill to be a part of this renaissance of elemental mass spectrometry.
Alexander Gundlach-Graham is a Research Scientist at ETH Zurich, Switzerland.
- A Praetorius et al, “Single-particle multi-element fingerprinting (spMEF) using inductively-coupled plasma time-of-flight mass spectrometry (ICP-TOFMS) to identify engineered nanoparticles against the elevated natural background in soils”, Environ Sci: Nano 4, 307–314 (2017).
Alexander Gundlach-Graham earned his Ph.D. in 2013 in the group of Gary Hieftje at Indiana University for the development and characterization of distance-of-flight mass spectrometry (DOFMS). Afterwards, he received a Marie-Curie Fellowship to complete postdoctoral research in the lab of Detlef Günther at ETH Zurich. Alex currently holds an Ambizione fellowship through the Swiss National Science Foundation. His research focuses on ICP-time-of-flight mass spectrometry and its application for high-resolution laser-ablation ICP-MS imaging and nanoparticle detection and sizing.