Applying Infrared Spectroscopy at the Nanoscale
How to meet today’s demands for nanoscale chemical analysis in materials and life sciences.
Infrared spectroscopy and microscopy rapidly analyze materials to create fingerprints that identify chemical species and create spatially resolved maps of chemical composition. Both techniques are used routinely in academic and industrial research settings but infrared microspectroscopy suffers from the limitation that, in practice, the spatial resolution is limited by optical diffraction to around 10-30 μm.
Extending IR spectroscopy beyond optical diffraction limits is a critical unmet need. Governments and industries have invested billions of dollars in developing novel materials and devices at nanoscale dimensions, far below the spatial resolution limit of conventional IR spectroscopy. And in the life sciences, conventional IR microspectroscopy has generally been limited to the scale of cells or larger, lacking the ability to perform chemical analysis at the sub-cellular level.
The recent combination of atomic force microscopy and IR spectroscopy (AFM-IR) offers a solution. Researchers have obtained IR spectra with spatial resolution somewhere between 50 and 500 times better than conventional Fourier transform IR microspectroscopy. The AFM-IR technique works by using the tip of an atomic force microscope (10-100 nm in diameter) as a nanoscale detector of local infrared light absorption.
In more detail, the AFM tip acts as a near-field detector of the photothermal expansion that occurs when IR laser pulses are absorbed by chemically-specific molecular vibrations in the sample. IR absorption spectra are created by measuring this photothermal expansion as a function of the wavelength of incident laser light. Chemical composition images can be obtained by mapping this photothermal absorption across a region of a sample with the laser tuned to an absorption band corresponding to a chemical species of interest. Because the photothermal expansion is detected locally with the AFM tip, the spatial resolution is not limited to the optical diffraction limit of conventional IR spectroscopy.
The implications of being able to apply the powerful chemical identification technique of infrared spectroscopy at the nano scale in the materials and life sciences are far-reaching. Recent publications using AFM-IR have encompassed materials fields such as polymer blends, composites and multilayers; electrospun fibers; pharmaceuticals; plasmonics; fuel cell membranes, and semiconductors. In engineering polymeric materials, for example, materials developers use multiple polymeric components and a wide range of additives (for example, carbon fibers, nanocrystalline clay, carbon nanotubes, graphene) to achieve the desired functional properties. AFM-IR is becoming a critical tool for the chemical characterization of material component distribution and the interphase region between polymer domains and various additives.
Meanwhile, in the life science fields, AFM-IR has been used to map triglyceride vesicles in bacteria for biofuel production, to reveal protein/mineral concentration in bone, and to map lipids in skin.
Recently, the sensitivity of the AFM-IR technique has been enhanced, making it possible to perform IR nanospectroscopy on samples down to a thickness of a single molecular monolayer and with a spatial resolution of around 25 nm. This has been demonstrated on both self-assembled monolayers and biological membrane samples.
Clearly, spectroscopy’s ability to chemically characterize samples in the materials and life sciences ultimately depends on the correct interpretation of the acquired spectra.
Fortunately, AFM-IR measures the same fundamental property as conventional IR spectroscopy, namely the amount of absorbed infrared light. As such, the same techniques used to analyze conventional FT-IR spectra are directly applicable to AFM-IR spectra. In fact, AFM-IR spectra have been used to reverse-engineer laminated polymer multilayer films and polymer blends by taking advantage of IR materials databases and conventional IR interpretation expertise.
However, in several cases AFM-IR spectra reveal features simply not visible in bulk spectra. Conventional IR spectroscopy averages over regions that are many microns to many millimeters across and the resulting absorption peaks may be broadened due to the many molecular orientations and local environments within a bulk sample. On the other hand, AFM-IR measures regions that are small enough to reveal chemical heterogeneity that is averaged out over larger length scales; this reveals local molecular orientation or shifts in band height/shape due to molecular interactions at sub-micron length scales.
Together, the sensitivity and new information afforded by improved spatial resolution are enabling AFM-IR to open a whole new window on the chemical morphology.
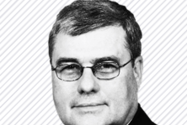
Curt Marcott worked for over 28 years as an infrared spectroscopist at Procter & Gamble after which, he joined the spectroscopic consulting firm Light Light Solutions. in 2007. “Although I was very proud of the role I played in development of IR spectral imaging and its application to industrial problems, P&G managers were always asking me what it would take to look at smaller objects. Now in a ‘retirement’ job, I am really enjoying helping to make that dream a reality.” Curt is also an adjunct professor at the University of Delaware and Miami University in Oxford, Ohio.
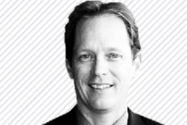
When I was a child, I was given a large magnifying glass. When I wasn’t trying to set fires with it, I spent a large amount of time looking at things that were too small for my unaided eyes to see,” says Craig Prater. “This passion has continued through my professional life, developing instrumentation to reveal fascinating structures.” Craig joined Anasys as CTO in 2007 to focus on nanoscale materials characterization.