CD-MS: “A Whole New Ballpark for Mass Spectrometry”
Evan Williams is surprised that more companies aren’t interested in commercializing charge-detection technologies, given their potential to address challenges in viral particle analysis, synthetic nanoparticle characterization, and single-cell analysis – areas where traditional methods fall short
James Strachan | | 8 min read | Interview
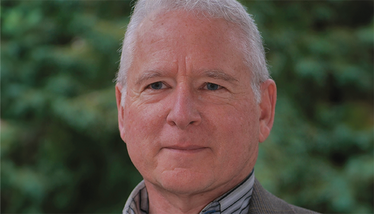
Image Credit: Catherine Siemens
When did you first become interested in charge detection mass spectrometry (CD-MS)?
I first became interested in this area well over 20 years ago when I attended an electrostatic ion trap workshop and saw some of the cone traps from Daniel Zajfman’s group at the Weizmann Institute of Science, Israel. They were being used for ion storage in rings, performing isolation and storage tasks. Unfortunately, it took us about eight years to secure funding to build our first instrument, which set us back considerably because it was essentially a ground-up build. Despite the challenges, we stuck with the cone traps, which is a bit different from Martin’s approach.
In an FTMS-type instrument – whether it’s an Orbitrap, an ICR, or these electrostatic ion traps – the frequency is always directly related to the mass-to-charge ratio (m/z). The idea is that by measuring a frequency, you can determine m/z. Most people prefer the frequency to remain stable so they can measure it over a long period, which allows for very precise mass measurements. Orbitraps and ICRs, for example, are known for their high accuracy in this regard.
However, in electrostatic ion trap devices, ion frequency is energy-dependent. Martin Jarrold’s strategy has been to try to minimize this energy dependence by designing harmonic traps. While you can never achieve a perfectly harmonic trap – there will always be some energy dependence – you can minimize it over a narrow range of energies.
In contrast, our approach involves trapping ions with energies ranging over 60-70 eV per charge, which is a significant range. The advantage of this with single-ion techniques is that if you have multiple ions with the same m/z, you can spread their energies out, so they each have different frequencies. We continuously track the m/z, charge, and ion energy throughout the entire time that an ion is trapped. This results in fewer interferences and allows for a much higher degree of multiplexing. We can introduce more ions into the device without their frequencies interfering or stacking up, which is a limitation in techniques like the Orbitrap. In the Orbitrap, if you fill it with ions of the exact same m/z, all their signals co-add, and you lose information about the number of ions or their charges. Our approach helps avoid this issue.
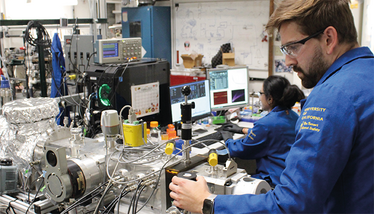
The team's CD-MS instrument. Foreground: Zachary Miller; background: Veena Avadhani. Photo taken by Jacob Jordan.
Are there any other main approaches in the field besides yours and Martin’s?
Those are the major approaches, but there's another researcher, John Hoyes, who has developed a figure-eight version of these traps. The advantage of his design is that it’s larger, allowing more ions to be trapped. However, the downside is that it currently only allows for the examination of very narrow m/z segments.
For us, we’re focusing on increasing the range of m/z that can be analyzed simultaneously. Additionally, we’re prioritizing speed, aiming to significantly reduce the time required for these measurements. Once you get into the million Dalton or mega-Dalton range, high resolution becomes less critical due to the inherent heterogeneity of the samples. For instance, when analyzing samples like an AAV (adeno-associated virus), while a commercial Orbitrap instrument is capable of very high resolution, the best spectra produced by that instrument actually have similar resolution to ours. This isn’t due to the instrument itself, but rather the heterogeneity of the samples.
Given this, we believe that focusing on high resolution becomes less important compared to other factors such as high ion transmission, significant multiplexing, and the ability to make measurements quickly – comparable to what you’d expect from a standard mass spectrometer. Currently, we typically measure spectra of samples in about one to five minutes, but if you want to couple this with LC, the measurement time needs to be reduced even further, ideally to a few seconds.
What other promising applications of CDMS are you exploring?
I would say this field is wide open right now because accurate mass measurement of very large particles, such as synthetic nanoparticles, has been challenging. We now have collaborations with several groups where CD-MS is proving to be the best, and sometimes the only, method for characterizing these particles. Traditionally, microscopy has been the primary tool, but it has its limitations. For example, techniques like TEM (transmission electron microscopy) require drying out the sample on a grid, which can introduce artifacts. Additionally, microscopy isn’t well-suited for obtaining kinetic information, as it typically involves pulling samples out at different times.
The advantage of CD-MS for large particles is similar to the advantage of conventional mass spectrometry for smaller molecules (below a MDa): it provides very accurate mass measurements, quickly, with high sensitivity and specificity. This is valuable for characterizing anything involving large particles, whether it’s viral particles, synthetic lipid nanoparticles, or other examples we’ve been working on. For instance, we can determine how much RNA is in lipid nanoparticles, what the average size of the particles is, and how they are affected by processes like freezing.
These samples are often very fragile, and while cryo-TEM is the standard technique for such analyses, it’s not always the most practical or rapid method. Mass spectrometry offers several advantages over microscopy in these contexts, especially for quick and practical analysis.
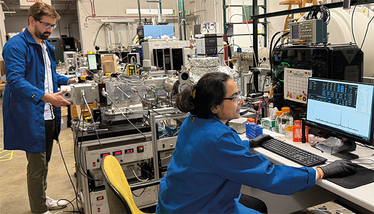
Foreground: Veena Avadhani; background: Zachary Miller. Photo taken by Evan Williams
Just how large can we go with CD-MS?
We’d like to be doing single-cell analysis right now, and there are many good reasons to pursue cellular analysis based solely on mass. However, once you get into the micron size range, other techniques might be more suitable. The challenge of heterogeneity becomes more significant at that scale, and high mass accuracy becomes less critical. For instance, with CD-MS, we are time-limited because it’s a Fourier transform technique – meaning the longer we measure a signal, the more accurate the mass measurement becomes.
Even with just 1 percent mass accuracy, which we can achieve in about 100 milliseconds of acquisition time, we’re talking about resolving fractions of a nanometer in terms of particle diameters. At some point, the mass accuracy is so high that it enables us to resolve down to a fraction of an angstrom, like a tenth of an angstrom in diameter resolution, but in practice, that level of precision is not likely to be useful.
For most problems in high-mass applications, something with reasonably good mass accuracy is sufficient. At larger size, you have other techniques like microbalances that take over, allowing you to analyze individual particles with devices like SAW (surface acoustic wave) sensors and other types of microanalysis tools.
How would you characterize the current state of CDMS – especially in relation to alternative techniques?
There are optical methods for single-particle analysis, which have some advantages. One of the key benefits of any solution-based technique is that you can typically work in buffer solutions that are biologically relevant. In contrast, most mass spectrometry of "native" particles is done in ammonium acetate, but biology doesn’t naturally operate in ammonium acetate.
For stable structures, this may not make a significant difference, but for most biological samples, people typically perform buffer exchanges from more common buffers like phosphate or Tris, which contain sodium chloride. Forming ions directly from these types of physiological buffers is important. For example, when working with lipid nanoparticles, we don’t perform a buffer exchange because they’re not stable in ammonium acetate, at least in our experience. Instead, we run them straight out of a phosphate buffer, which closely mimics the environment of a buffer that might be used to inject patients. Many other solution-phase techniques do the same.
I would say that a major obstacle for mass spectrometry in general is the reliance on non-physiological buffers, like ammonium acetate, and the need to switch to more biologically relevant conditions. The reason this hasn’t been done more widely is that the salts in these physiological buffers tend to stick to ions in the gas phase, leading to artificially high masses and broad peaks. However, for very large particles, we don’t see this as a significant issue because the need for high mass accuracy and resolution diminishes as the particle size increases.
What are the current limitations of CD-MS?
We’re starting to learn a lot more about the technique, particularly with electrospray ionization, which is how most ions are introduced into the instrument. Getting large particles through electrospray, at least the way we currently do it, is likely to be a significant challenge. While we haven’t hit the limits yet, we believe this area will require further development.
Another significant issue is the speed of the devices. A lot of the data being published involves long accumulation times – sometimes an hour or two just to get a decent ion count. That’s acceptable when you’re developing a new technique, but in practical applications, it’s not something most users are willing to endure. Our goal is to reduce this time to be more in line with an LC time scale.
What does the commercial landscape for CD-MS look like?
Martin started a company, and I believe Waters has licensed the technology from him and is in the process of trying to commercialize it. Thermo has already commercialized the Orbitrap version of single-ion mass spectrometry, which has been a commercial product for at least two years now. They’re using an established instrument in a new way, but I haven’t seen any very high-mass data from Thermo’s instrument.
As for the devices we’re currently developing, I’m actually surprised that more companies aren’t interested in commercializing them. This technology really does open up a whole new ballpark for mass spectrometry.
Evan Williams is Professor of Chemistry at UC Berkeley, USA

Over the course of my Biomedical Sciences degree it dawned on me that my goal of becoming a scientist didn’t quite mesh with my lack of affinity for lab work. Thinking on my decision to pursue biology rather than English at age 15 – despite an aptitude for the latter – I realized that science writing was a way to combine what I loved with what I was good at.
From there I set out to gather as much freelancing experience as I could, spending 2 years developing scientific content for International Innovation, before completing an MSc in Science Communication. After gaining invaluable experience in supporting the communications efforts of CERN and IN-PART, I joined Texere – where I am focused on producing consistently engaging, cutting-edge and innovative content for our specialist audiences around the world.