Exploring Chirality in Outer Space
What is the origin of stereochemical bias – terrestrial autocatalytic processes, extraterrestrial contamination or otherworldly intervention? Here, I review the gas chromatographic tools being used in the search for homochirality in space – the final front
Volker Schurig |
Dedicated to the late Professor Emanuel Gil-Av - the pioneer of modern enantioselective chromatography - on the occasion of his centenary in 2016.
Stereochemical bias is considered to be one of the preconditions for the formation of life on Earth. Yet even in our third millennium, we do not know how the preference of the image over its incongruent mirror image was achieved – a phenomenon called molecular homochirality, single-handedness or symmetry-breaking. It is also unclear why evolution exclusively selected L-amino acids and D-sugars as homochiral building blocks of proteins and nucleic acids in all living species, including viruses, bacteria, plants, animals and humans.
The discrimination of chiral biogenic molecules, called enantiomers, may have occurred on Earth by autocatalysis, or may be the result of extraterrestrial contamination with homochiral molecules (caused by the existence of circular-polarized light in interstellar space) or, less likely in my opinion, as a result of parity-violation energy differences. Therefore, various space missions are under way – or are planned – to detect extraterrestrial homochirality. For this challenge, enantiomers, which possess strictly identical (nonchiroptical) properties in a nonchiral symmetric environment, must be resolved. Consequently, the current Rosetta- and Exo-Mars-missions are equipped with enantioselective gas chromatographic columns containing chiral stationary phases (CSPs) to separate and detect volatile enantiomers as biomarkers of life (1, 2).
Breakthroughs in enantioselectivity
The first direct separation of enantiomers by gas chromatography (GC) was described by Emanuel Gil-Av and coworkers in the year 1966 (see Figure 1). They resolved enantiomers of the N-trifluoroacetyl-O-alkylesters of the proteinogenic α-amino acids alanine, valine and leucine on a glass capillary column (100 m x 0.25 ID) coated with the optically-active CSP, N-trifluoroacetyl-L-isoleucine-O-lauryl ester (3). Scientists of the Weizmann Institute in Israel subsequently – and efficiently – mimicked the selective peptide-enzyme interaction based on hydrogen-bonding (4, 5). In 2016, we will celebrate the 50th anniversary of the development of modern chromatographic enantiomeric separation. The Gil-Av approach was consequently used to investigate extraterrestrial material; however, no amino acids were found (at a detection limit of 0.1 ppm) by NASA in lunar samples from the Sea of Tranquility (6).
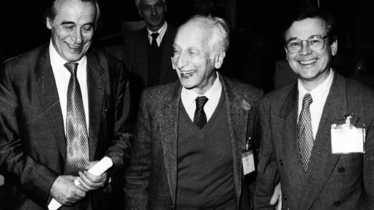
Figure 1. Three early promoters of enantioselective GC. Ernst Bayer (left), Emanuel Gil-Av (middle) and Volker Schurig (right) at the 3rd International Symposium on Chiral Discrimination, Tübingen, Germany, 1992.
Another concept for chirality recognition in GC in the realm of metal organic chemistry was also developed at the Weizmann Institute, where the enantiomers of the olefin 3-methylcyclopentene could be resolved on optically-active CSP dicarbonyl-rhodium(I)-3-trifluoroacetyl-(1R)-camphorate (7). The subsequent use of metal(II) ions started the development of chiral complexation GC for the enantiome ric study of chiral pheromones, flavors, fragrances, as well as oxiranes formed by enantioselective epoxidations and on-column enantiomerizations of configurationally labile racemates (8).
A third – nearly universal – tool in enantioselective GC is the use of alkylated/acylated cyclodextrins coated on high-resolution fused silica capillary columns (9, 10). Almost all classes of volatile chiral compounds can be resolved, including unfunctionalized saturated hydrocarbons devoid of any chemical functionality, such as the racemic methylethyl(i)propylmethanes C*HMeEtPr and C*HMeEtiPr (11). In particular, the resolution of chiral alkanes commands interest of the forthcoming search for extraterrestrial homochirality (12). The Cassini-Huygens mission on Saturn’s moon Titan detected methane and higher hydrocarbons and chiral entities may either be racemic or enantiomerically enriched.
A breakthrough in enantioselective GC was achieved by Frank, Nicholson and Bayer (see Figure 1) at Tübingen University when they chemically linked the chiral selector N-acyl-L-valine t-butylamide (5) to polydimethylsiloxane (see Figure 2) thus combining the enantioselectivity of valine with the universal gas chromatographic properties of liquid silicones. On the CSP Chirasil-L-Val (coated on an 18 m x 0.3 mm ID glass capillary column) 17 proteinogenic α-amino acids could be simultaneously enantioseparated as N-pentafluoropropanoyl-O-isopropyl esters in less than 30 min (13). All L-amino acids are eluted as the second fraction, but the elution order can be reversed by employing Chirasil-D-Val. Chirasil-Metal (see Figure 2, middle) can be used as the CSP in GC and supercritical fluid chromatography (SFC) (14).
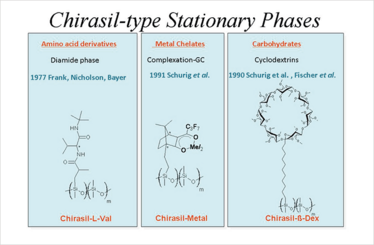
Figure 2. Development of Chirasil-type stationary phases for enantiomeric separations by GC.
Investigating enantiomeric excess
In Chirasil-β-Dex, permethylated β-cyclodextrin is chemically bonded via an octamethylene spacer on polydimethylsiloxane (see Figure 2, right) (14). Its temperature range extends from -10°C to 250°C. One single column (80 cm x 0.5 mm ID) coated with thermally immobilized Chirasil-β-Dex can alternatively be used in GC, SFC, liquid chromatography (LC) and capillary electrochromatography (CEC) for the same racemic compounds (in other words, it’s a unified approach) (15). In samples from the Murcheson- and Murray meteorites, Chirasil-β-Dex has been employed to detect enantiomeric excess of up to 15.2 percent for L-isovaline (2-amino-2-methyl-butyric acid) (16). The extraterrestrial origin of the enantiomeric bias has been proved using isotopic analysis by GC-combustion isotopic ratio mass spectrometry (GC-C-IRMS) (16).
Chirasil-β-Dex has also been used to correlate dextro-rotatory glyceraldehyde, the key reference compound of stereochemistry, with the absolute configuration of R,R-trans-2,3-dideuterooxirane determined by the novel method of Coulomb explosion imaging (17). Emil Fischer’s arbitrary assignment of D-configuration for (+)-glyceraldehyde was thus confirmed once and for all: sugars are D-configurated and α-amino acids are L-configurated on Earth!
In the binary CSP Chirasil-Val-Dex, two complementary selectors are attached to polydimethylsiloxane, allowing a broad enantioseparation spectrum that includes racemic hydrocarbons and derivatized 2-amino acids, which are simultaneously enantioseparated (see Figure 3) (18).
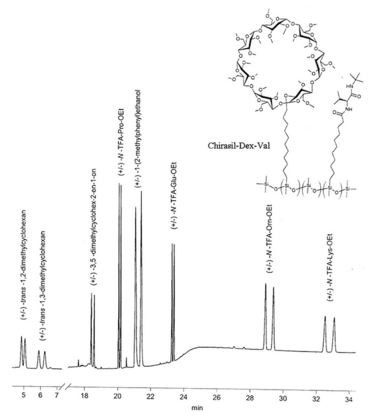
Figure 3. Simultaneous GC enantiomeric separations (signal ratio 1:1) of racemic cycloalkanes, a ketone, an alcohol and the 2-amino acids proline, glutamic acid, ornithine and lysine (as N-trifluoroacetyl-O-ethyl esters) on Chirasil-Dex-Val (insert) on a fused silica capillary (20 m x 0.25 mm ID x 0.25 μm CSP layer) with temperature program and dihydrogen as carrier gas (18).
Where no man has gone before
The European Space Agency (ESA) Rosetta mission and the landing of the robot Philae on the comet 67P/Churyumov-Gerasimenko (nicknamed Chury, pictured on opening page) was awarded ‘Breakthrough of the Year 2014’ by the American Association for the Advancement of Science (19). The comet Chury is considered to be a remainder from the formation of the solar system 4.6 billion years ago – and as such, it may serve as a model of primordial Earth for bio-astronomers.
As a note for history enthusiasts, the ambitious mission by ESA was named after the inscription-covered Rosetta stone and the temple obelisk Philae, which helped Jean-Françoise Champollion to decipher the Egyptian hieroglyphs in 1822.
Rosetta was launched on March 2, 2004 and Philae landed on the comet on November 11, 2014 at a distance of 510 million kilometers from Earth – signal transmission at that distance takes about half an hour! The Rosetta orbiter and Philae lander are equipped with ten miniaturized measuring devices endowed with low energy consumption.
To detect enantiomerically enriched chiral molecules in interstellar space, GC is the method of choice. It is devoid of liquid mobile phases, instrumentation is simple and shock-resistant, the combination with mass spectrometry (GC-MS) is legion, miniaturization is feasible and due to the enormous separation power of high-resolution capillary GC, multicomponent analysis is straightforward.
GC-MS at 38 km/s (truly fast GC)
In fact, Philae contains two GC-MS systems. The British project, Ptolemy, employs three capillary columns (you can read a little more about its inception here: tas.txp.to/1215/Ptolemy), but I’d like to focus on the Cometary Sampling and Composition (COSAC) project, which was developed at the Max-Planck-Institute for Solar System Research, Göttingen, Germany. COSAC is based on a GC-time-of-flight (TOF)-MS system (with a mass range of 1-1500 amu), which can analyze samples delivered by the sample drilling and distribution system (SD2) (20, 21). COSAC can also operate in a “sniffing mode”, in which the MS accumulates data without active sampling from SD2. COSAC employs a sampling oven heated up to 180°C and a pyrolysis oven, which can reach temperatures of 600°C. The gases originating from the heated samples are analyzed utilizing eight 10–15 m capillary columns connected in parallel, three of them coated with CSPs. The GC uses helium as the carrier gas (refined valve connections are used to keep the helium consumption at a minimum) and is coupled to a thermo conductivity detector.
It was reported in July 2015, that 25 minutes after Philae’s initial contact with the cometary nucleus, COSAC carried out its first chemical analysis in the sniffing mode by examining particles that were passively entering the instrument. Sixteen compounds were identified, divided into six classes of organic molecules (alcohols, carbonyls, amines, nitriles, amides and isocyanates). Of these, four were detected for the first time on a comet (methyl isocyanate, acetone, propionaldehyde and acetamide) (22). Investigators concluded, “the complexity of cometary nucleus chemistry and the importance of nitrogen-containing organics imply that early solar system chemistry fosters the formation of prebiotic material in noticeable concentrations...” (22).
Specifically for chirality experiments, COSAC was equipped with three fused silica columns coated with CSPs (20, 21, 23), see Figure 4. Chirasil-L-Val was chosen for chiral α-amino acids and Chirasil-β-Dex was selected for chiral hydrocarbons. Non-bonded 2,6-O-dipentyl-3-O-trifluoroacetyl-y-cyclodextrin (CD-G-TA) (24) was selected for unspecified compounds. In contrast to Chirasil-L-Val, the cyclodextrin selectors are not prone to radio-racemization (25). Unfortunately, native amino acids are involatile and have to be derivatized prior to GC analysis. The traditional two-step protocol to form N-perfluoroacyl-O-alkyl esters appeared unsuitable for space experiments. Hence, the one step reaction with N,N-dimethylformamide/O,O-dimethylacetal (DMF-DMA) (see Figure 5) was developed (26). Notably, only valine, isoleucine, aspartic acid and phenylalanine can be resolved using this method. The Chirasil-β-Dex column was operated shortly after Philae’s touchdown on Chury (Uwe Meierhenrich told me), but no data have been disclosed by ESA. Subsequent measurements will only be possible when the batteries of Philae – the lander, which is currently lying dormant – are recharged at an elevated solar altitude. No news on this topic is currently forthcoming...
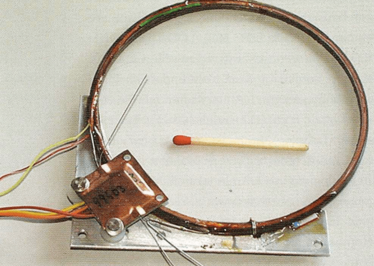
Figure 4. Fused silica capillary column including heating device and thermo conductivity detector as installed on the chirality module of the COSAC instrument onboard Rosetta’s lander Philae (Evans). Courtesy of U. J. Meierhenrich.

Figure 5. Derivatization of α-amino acids with DMF-DMA (26).
To Jupiter... and beyond?
Polymeric Chirasil-β-Dex is insensitive to radiation existing in interstellar space and is considered to be the optimal CSP to detect homochirality on Mars, being exclusively applied for current Mars-science laboratory (MSL) missions (27). Exo-Mars 2018 is a joint mission by the ESA and the Russian Federal Space Agency (Roskosmos) – but the gas chromatography element is being provided by the French space agency, CNES. CNES’ Mars Organic Molecule Analyser (MOMA) uses Chirasil-β-Dex in a stainless-steel capillary column and uses helium as the carrier gas and also contains a DMF-DMA kit for the derivatization of amino acids and hydroxycarboxylic acids (26). Chirasil-β-Dex CSP has also been proposed for GC experiments in the planned NASA mission to Jupiter’s moon, Europa.
Whether robotic chirality-experiments in space will succeed is still a matter of conjecture, especially given that the resolution of mirror-image molecules represents a non-trivial task even in earthbound and manned laboratories! But given that our astronautic activities may lead to contamination of the universe with man-made organic material, mass spectrometric isotopic analysis will be required to prove the foreign origin of molecules detected in comets, moons and planets. Whatever the outcome, I believe that the challenge of performing chirality experiments in interstellar space is at least likely to stimulate important terrestrial applications!
Note: fused silica columns coated with Chirasil-β-Dex were first commercialized by Chrompack International, Middleburg, The Netherlands. The brand was later transferred to Varian Inc. and finally to Agilent Technologies.
- UJ Meierhenrich, “Comets and Their Origin – The Tool to Decipher a Comet”, Wiley-VCH, Weinheim, 2015. ISBN: 978-3-527-41281-5
- I Myrgorodska et al., “Molecular chirality in meteorites and interstellar ices, and the chirality experiment on board the ESA Cometary Rosetta Mission”, Angew Chem Int Ed 53, 2-13 (2014). DOI: 10.1002/anie.201409354 (Minireview dedicated to Volker Schurig).
- E Gil-Av, B Feibush, R Charles-Sigler, “Separation of enantiomers by gas liquid chromatography with an optically active stationary phase”, Tetrahedr Lett 7, 1009-1015 (1966).
- E Gil-Av,“Present status of enantiomeric analysis by gas chromatography“, J Mol Evol 6, 131-144 (1975).
- B Feibush, “Chiral separation of enantiomers via selector/selectand hydrogen bondings”, Chirality 10, 382-395 (1998).
- J Oró et al., “Organogenic elements and compounds in surface samples from the Sea-of-Tranquility”, Science 167, 765-767 (1970).
- V Schurig, E Gil-Av, “Chromatographic resolution of chiral olefins. Specific rotation of 3-methylcyclopentene and related compounds“, Isr J Chem 15, 96-98 (1976/77).
- V Schurig, “Practice and theory of enantioselective complexation gas chromatography”, J Chromatogr. A 965, 315-356 (2002).
- V Schurig, H-P Nowotny, “Gas chromatographic separation of enantiomers on cyclodextrin derivatives", Angew Chem Int Ed 29, 939 -1076 (1990).
- WA König, “Gas Chromatographic Enantiomer Separation with Modified Cyclodextrins”, Hüthig, Heidelberg, 1992.
- V Schurig, D Kreidler, “Gas-chromatographic enantioseparation of unfunctionalized chiral hydrocarbons: an overview”, In: Methods in Molecular Biology, Vol. 979. DOI: 1007/978-1-62703-263-6 3, Springer Science, 2013.
- UJ Meierhenrich et al., “Enantiomer separation of alkanes in preparation of ROSETTA’s ‘Chirality Experiment’”. Chirality 13, 454-457 (2001).
- H Frank, GJ Nicholson, E Bayer, “Rapid gas chromatographic separation of amino acid enantiomers with a novel chiral stationary phase”, J Chromatogr Sci 15, 174-176 (1977).
- V Schurig, D Schmalzing, M Schleimer, “Enantiomer separation on immobilized Chirasil-Metal and Chirasil-Dex by gas chromatography and supercritical fluid chromatography”, Angew Chem Int Ed 30, 987-989 (1991).
- V Schurig et al., “Toward unified enantioselective chromatography with a single capillary column coated with Chirasil-Dex”, Angew Chem Int Ed 33, 2222-2223 (1994).
- S Pizzarello, M Zolensky, KA Turk, “Nonracemic isovaline in the Murcheson Meteorite: Chiral distribution and mineral association”, Geochim et Cosmochim Acta 67, 1589-1595 (2003).
- Zawatzky et al., “Coulomb explosion imaged cryptochiral (R,R)-2,3-Dideuterooxirane: unambiguous access to the absolute configuration of (+)-glyceraldehyde”, Chem Eur J 20, 5555-5558 (2014). DOI: 10.1002/chem.201400296
- PA Levkin, A Levkina, V Schurig, “Combining the enantioselectivities of L-Valine diamide and permethylated β-cyclodextrin in one gas chromatographic chiral stationary phase”, Anal Chem 78, 5143-5148 (2006).
- E Hand, “Comet Breakthrough of the Year & People’s Choice”, Science 346 (6216), 1442-1443 (2014). DOI: 0.1126/science.346.6216.1442
- F Goesmann et al., “COSAC, the Cometary Sampling and Composition Experiment on PHILAE” Space Sci Rev 128, 257-280 (2007). DOI: 10.1007/s11214-006-9000-6
- C Szopa et al., “The COSAC Experiment of the Rosetta Mission: performance under representative conditions and expected scientific return”, Adv Space Res 40, 180-186 (2007). DOI: 10.1016/j.asr.2007.04.086
- F Goesmann et al., “Organic compounds on Comet 67P/Churyumov-Gerasimenko revealed by COSAC mass spectrometry”, Science 349 (6247) (2015). DOI: 10.1126/science.aab0689.
- AC Evans et al.,“The ROSETTA mission and the COSAC experiment”, In: Differentiation of Enantiomers II (V Schurig, ed.), Top Curr Chem 341, 271-300 (2013) (Chapter 4). DOI: 10.1007/128_2013_442
- A Berthod, W Li, DW Armstrong, “Multiple enantioselective retention mechanisms on derivatized cyclodextrin gas chromatographic chiral stationary phases”, Anal Chem 64, 873-879 (1992).
- O Trapp, V Schurig, “Interconversion of chiral molecules in interstellar space”, Enantiomer 6, 193-194 (2001).
- C Freissinet et al., “Search for evidence of life in space: Analysis of enantiomeric organic molecules by N,N-dimethylformamide dimethylacetal derivative dependant gas chromatography-mass spectrometry”, J Chromatogr A 1217, 731-740 (2010).
- C Freissinet et al., “Enantiomeric separation of volatile organics by gas chromatography for the in situ analysis of extraterrestrial materials: kinetics and thermodynamics investigation of various chiral stationary phases”, J Chromatogr A 1306, 59-71 (2013).
Institute of Organic Chemistry, University of Tübingen, Germany.