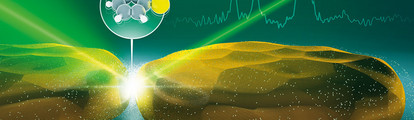
Gurus of Raman Spectroscopy
A group from the École polytechnique fédérale de Lausanne in Switzerland has proposed a potentially disruptive new model – dynamical backaction amplification (DBA) of molecular vibrations – to explain unexpected observations in surface-enhanced Raman spectroscopy (SERS). And they believe the work will open up opportunities for novel systems that further enhance the detection capability of SERS. However, the model has not been fully accepted by the spectroscopy community, with other researchers heading in different directions. Volker Deckert (University of Jena and Leibniz Institute of Photonic Technology, Germany) and Duncan Graham (University of Strathclyde and Renishaw Diagnostics, UK) offer their own thoughts on the future of Raman spectroscopy.
The DBA Lowdown
The set of vibrational frequencies of a molecule constitutes its unique fingerprint. Vibrational modes that are “Raman-active” interact with incident laser light in an inelastic scattering process. This results in secondary photons with a frequency shifted from the incident ones by the vibrational frequency. Raman spectroscopy leverages this process to optically measure the vibrational spectrum of a molecule (or a material) and thus reveal its chemical identity.
Unfortunately, Raman scattering has a very small cross-section and it is impossible to detect single molecules with a conventionally focused laser beam. A route to overcoming this limitation was discovered in 1997 independently by K. Kneipp et al. and S. Nie and S. R. Emory (2)(3). Using surface-enhanced Raman scattering (SERS), the teams dispersed individual molecules on rough metallic surfaces, which served to focus light down to molecular dimensions. The incident light generates localized oscillating electric fields in the metal, which are called plasmons. With the rapid progress of micro- and nano-fabrication techniques, researchers are now able to tailor the properties of these plasmons in a variety of metallic nanostructures, which has led to rapid improvements in sensitivity and unexpected observations such as nonlinear effects and anomalously large enhancement factors, which could not find explanations within the conventional models of SERS.
Christophe Galland
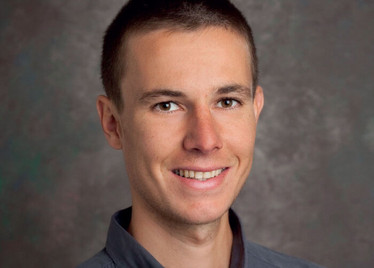
Christophe obtained his PhD from Ataç Imamoglu’s group at ETH Zürich (Switzerland) in 2010. He then spent two years as a postdoctoral fellow at Los Alamos National Lab (New Mexico, USA) with Victor Klimov, where he developed a novel spectroscopic technique to study the fluorescence of single nanocrystal quantum dots in an electrochemical cell. In 2012, he joined Michael Hochberg and Tom Baehr-Jones at the University of Delaware (USA) to implement quantum optical devices and experiments with silicon photonic integrated circuits. Since mid-2013, he has been the holder of a fellowship from the Swiss National Science Foundation in Tobias Kippenberg’s group. Christophe’s current research with PhD student Philippe Roelli focuses on the theory and experimental realizations of optomechanical systems based on molecules coupled to plasmonic cavities.
A different angle
Our group has an atypical approach to Raman scattering, since our expertise is not in molecular or crystal spectroscopy but rather in cavity optomechanics. Our field deals with carefully engineered micro-fabricated systems in which a mechanically compliant element modulates the resonance frequency of a high-quality optical cavity. When a laser excites such a system, a Raman process can generate or annihilate one quantum of mechanical excitation (that is to say, a phonon), in analogy to what happens in a molecule or a crystal. Yet the oscillation of optomechanical systems are typically at much lower frequencies and feature much lower damping rates and much longer relaxation times.
From our perspective, Raman scattering in optomechanical cavities is thus a tool to manipulate mechanical motion with light. In particular, you can optically damp mechanical oscillations, a technique that has been used in quantum optics to cool trapped atoms or ions to their motional ground state. The work we are doing, therefore, opens a new way to investigate molecular vibrations in the quantum regime using the concepts of quantum optics.
In our latest theoretical research, we developed a radically novel framework to model the plasmon-enhanced Raman interaction and calculate the Raman signal. We demonstrate that a vibrating molecule coupled to a localized plasmon is formally equivalent to an optomechanical cavity, a generic system in which a mechanical oscillator modulates the resonance frequency of an optical cavity, consisting of, for example, two facing mirrors. The radiation pressure of photons bouncing on the mirrors can be used to optically amplify their oscillating motion in a process called dynamical backaction amplification (DBA).
Our research shows that DBA can be used in SERS to amplify Raman-active molecular vibrations and thus provide a new mechanism for signal enhancement. In other words, light is more than a passive analytical tool; it also acts on the internal molecular degrees of freedom and drives molecular vibrations. The light-induced force, analogous to radiation pressure on a mirror, can thus be used to excite a particular vibrational mode of a molecule well above its thermal motion. The extra excitation greatly enhances the Raman signal, which is proportional to the vibrational energy.
Tobias Kippenberg
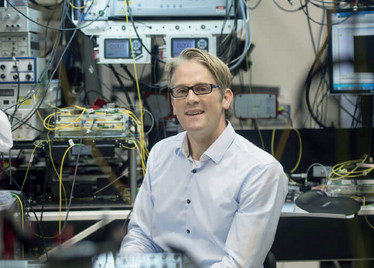
Tobias Kippenberg has been a full professor in the Institute of Condensed Matter Physics and Electrical Engineering at EPFL, Switzerland since 2013. Prior to EPFL he was Independent Max Planck Junior Research group leader at the Max Planck Institute of Quantum Optics (MPQ), Garching, Germany, in T. W. Haensch’s laser spectroscopy division. There he demonstrated radiation pressure cooling of optical micro-resonators, and developed techniques with which mechanical oscillators can be cooled, measured and manipulated in the quantum regime that are now part of cavity quantum optomechanics. His group also discovered the generation of optical frequency combs using high Q micro-resonators. Tobias is the recipient of several awards, including the EFTF Award for Young Scientists (2011), the Helmholtz Prize in Metrology (2009), the EPS Fresnel Prize (2009), ICO Award (2014), the Swiss Latsis Prize (2015), the Wilhelmy Klung Research Prize in Physics (2015) and he won first prize in the 8th European Union Contest for Young Scientists in 1996.
The implications?
The practical consequence of our findings is to question some widely accepted guidelines used to optimize the substrates and excitation schemes in SERS. Until now, researchers have obtained large signal enhancement by using broad plasmon resonances, which overlap with both the incoming laser and the outgoing Raman light. Considering DBA, we find two counter-intuitive conditions for obtaining amplification that is even more efficient. First, one should strive for narrower plasmon resonances (more precisely, the width of the resonance should be smaller than the vibrational frequency). Second, the excitation laser should be blue-detuned from the plasmon by exactly the vibrational frequency of the mode to be amplified.
Following these new guidelines, researchers and engineers should be able to design and fabricate a new generation of plasmonic devices to push the detection limits of SERS and its analytical capabilities even further.
The frequency shift between the laser and the plasmon resonance determines which particular vibrational mode is amplified, which opens a route toward higher resolution spectroscopy and mode-specific amplification. By applying our method, it should be possible to drive one particular vibrational mode far out of thermal equilibrium, and thereby study the dynamics at a molecular scale, quantifying the degree of anharmonicity (deviation of a system from harmonic oscillation) of each mode and their inter-mode couplings.
In practice, if the conditions for efficient DBA can be achieved in a commercial device, it would enable very specific detection of given types of molecules by carefully choosing the laser wavelength with respect to the plasmon resonance. Moreover, this parameter is easily tunable, allowing the device to be reconfigured for different molecules. On the detection side, if mode-specific amplification is reached, you could dispense with using high resolution spectrometers to distinguish between different modes, as a single frequency should be amplified and contribute to the signal.
Exploring C. V. Raman’s Nobel Legacy
What is your current research focus?
Volker Deckert: I’ve been working with Raman spectroscopy since my diploma thesis and I’ve always appreciated the different directions that this technique allows one to move in. Even though more and more commercial systems are available, ranging from scientifically flexible systems to handheld systems for all kind of applications, there is still space for instrumental progress in the academic environment. Such instrumental development has been always one of my favourite areas. Improving detector and spectrometer capabilities, developing difference spectroscopy for multichannel detection, adapting spectrometers for “terahertz Raman” spectroscopy before it was even given the name – I loved doing that. Certainly this playing (fooling?!) around was an important aspect when developing near-field Raman systems and TERS. The latter two are most probably the most defining techniques for me and my group and continue to challenge us even after all this time. Pushing the lateral resolution limit of Raman spectroscopy was and still is my favorite subject. Currently, my work has moved towards more applied scientific cases and the challenge is shifting from the instrumental details towards sample preparation, data evaluation and even theoretical issues. Still, we’ve seen several surprises in recent years and I am looking forward to the next few!
Duncan Graham: We use chemistry to functionalize nanoparticles and design new methods of analysis based on surface-enhanced Raman scattering (SERS). We have a heavy emphasis on the role of the surface chemistry to enable the controlled enhancement of the Raman scattering and have developed a number of unique assays based on the properties of nanoparticles combined with SERS. There is a heavy emphasis on the analytical performance of our assays and we have been interested in the detection of biomolecules, such as DNA, proteins, and small biomarkers, as well as looking at cells, tissue and in vivo systems. We have also covered areas of interest in security applications (for example, trace detection of explosives), but our main focus is on life science and clinical applications.
Additionally, we work with the end users of our analytical techniques and breakthroughs from an early stage in terms of designing projects and attempting to translate them into actual use. To date, our lead example is a high-throughput assay for fungal infections using SERS; the research took place a few years ago but it is now being commercialized (through Renishaw Diagnostics Ltd) and has just been awarded CE marking for use in European hospitals. It proves that SERS can be used in a bioanalytical assay in a clinical setting if there is enough understanding of the underlying chemistry to obtain suitable analytical performance.
What are the main recent Raman milestones?
VD: The typical timeline begins with the application of the laser in the 1960s – and electronic recording more or less at the same time. In the 1970s and 1980s, the advent of Raman microscopes and multichannel detectors. All these technologies – and many more “small” developments – have helped to improve measurement time by orders of magnitude, changing acquisition times from hours and even days to sometimes only a few seconds. Modern Raman microscopes allow the acquisition of large areas so quickly that adaptation for clinical analysis is on the near horizon. Here, the intrinsic advantage of Raman as a label-free technique might change the way how diagnosis is being done in the future (either because of replacement with Raman or by pushing competing techniques to be developed further).
Particularly important to my favorite technique – near-field Raman – was of course the discovery of surface-enhanced Raman spectroscopy (SERS) effect by Fleischmann, van Duyne and Creighton in the 1970s. Indeed, SERS is a branch of Raman spectroscopy that is still a driving force for innovations in the field. A milestone was definitely the discovery of single molecule sensitivity in the 1990s and beginning of the new century by Nie, Kneipp, Etchegoin and again van Duyne. With the advent of tip-enhanced Raman spectroscopy (TERS) in 2000, the basic principles of SERS allowed a confinement of the lateral resolution far beyond the diffraction limit. All these advances culminated in the recent detection of sub-molecular units by Dong et al and Deckert et al. And though the reason behind the increased resolution is still controversial, experiments clearly indicate sub-nanometer resolution, which is, in turn, driving theoretical developments to explain the facts.
DG: The big milestones in Raman have been written about in several reviews by other authors ((4), for example), but they tend to feature breakthroughs that are fundamental rather than applied. In terms of applications, Shuming Nie’s first paper on the use of SERS for in vivo analysis in 2008 prompted a lot of subsequent activity (5). Another game-changer is Pavel Matousek (Rutherford Appleton Laboratory) who has been developing spatially offset Raman scattering for security applications. His work has now moved onto the analysis of living systems where he can penetrate deep into tissues (6). From a personal perspective, our biggest breakthrough was in 2008 when we showed that a nanoparticle assembly could be used to turn on the enhancement of Raman scattering using biological interactions. That work has now been expanded into a variety of studies (7). And, more recently, a collaborative paper with Ewan Blanch at the Royal Melbourne Institute of Technology, Australia, has shown the first through space transfer of chirality in surface-enhanced Raman optical activity, which has implications for the study of the chirality of biomolecules at low concentrations (8).
Volker Deckert
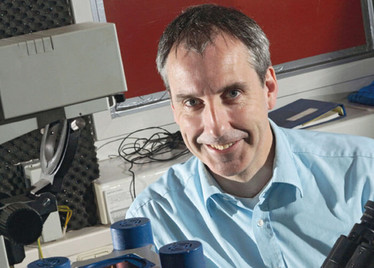
Volker Deckert is the head of the “Nano Spectroscopy Group” at the Institute of Physical Chemistry, University of Jena, Germany. The Group’s research goal is to push the lateral resolution of vibrational spectroscopy. The main tool for related projects is tip-enhanced Raman scattering (TERS). Current research with TERS includes biopolymer sequencing, fundamental reactivity on plasmonic assisted reactions, membranes and membrane proteins (studying the nanoscale composition of membranes with respect to lipid distribution and inclusion of proteins, shedding light on intermolecular and intramolecular interactions of protein structures, and examining the basic mechanism of drug delivery), and fast and efficient diagnostics of viruses to establish an ultra-sensitive technology for the qualitative analysis of different viruses and their specific antibodies.
Where does Raman sit in the world of chemical analysis?
DG: Raman is being used a lot at the moment in terms of chemical analysis. It is gaining a position in process analytical technology for online analysis of chemical reactions with feedback loops to allow improvement of scaled up chemical synthesis. I think analytical chemists are very aware of the potential for Raman scattering to be used in various scenarios; however, the weak scattering from a number of molecules tends to limit this technique to bulk analysis. In terms of trace analysis, advanced techniques such as SERS and TERS can play a role and they are starting to gain traction in the chemical and bioanalytical sectors. Reproducibility can be an issue and I believe that this can be achieved for SERS, and also for TERS with appropriate (and sufficient) research and effort.
VD: The number of instrument makers increases at every exhibition, so it seems that more and more applications arise. My guess is that those applications are mostly analytical. And if such applications reach a routine stage they might be extremely successful; however, from an academic point of view those applications are not necessarily in the focus of research and might be less visible, for instance, at conferences. The number of attendees at the recent International Conference on Raman Spectroscopy, clearly indicates an increasing interest in the field both in academia and in industry. In areas where the science case is still close to the potential application (for instance, bio-medical research), I dare say that Raman is getting more and more attention on the clinical side. So I can see that the analysis in this field will profit from the benefits of Raman spectroscopy, but because of elaborate medical trials, it will certainly take several more years until Raman becomes as commonplace as it is, for example, in geology.
How do you see the role and impact of SERS developing?
DG: The main challenge for SERS is to find the right application for the technique where it offers something beyond other analytical techniques. There are a number of two and three-dimensional surfaces commercially available that are interesting from a research perspective, but I am not aware of a killer application for nano-structured surface-based SERS technology, despite there being a growing number of vendors. In terms of solution- or suspension-based approaches where nanoparticles are used, SERS is gaining an advantage over other techniques. And I believe that this will move into the chemical and bioanalytical sectors. Again, the technical challenge really lies in the reproducibility that comes from having a solid and in-depth understanding of the surface chemistry; everything has to adsorb on the surface to be enhanced. Once you can control this, you get reproducible SERS. Much is said about having the right substrate and the right particles, which is of course important, and you can make nanoparticles very reproducibly, which will give a consistent enhancement – but only if your surface chemistry is robust and reproducible. This has an impact on imaging where enhancement from nanoparticles needs to be reproducible and similar, in particular, when looking at biological systems. There is a lot of work going on in this sector right now and I think this is an area of great interest for the SERS community. The effects will be felt in the life science and clinical sectors.
VD: Wherever high sensitivity is required, SERS is a potential option. At the moment, there is a big discrepancy between laboratory substrates that can reach extremely high enhancement factors and commercially available substrates that show, in general, much lower enhancement, but are reliable and potentially also comparable. Some consolidation is required along with the understanding that high enhancement factors usually come at a cost. I am sure that SERS in combination with handheld instruments will have a big share in the market when sensitive qualitative analysis is required. Quick tests for drugs could be an example. Solving the quantitative issue seems to me much more challenging, but in combination with lab-on-the-chip technologies this challenge can probably be solved as well.
From an imaging point of view, SERS imaging is somewhat comparable to MALDI imaging. As a substrate has to bind onto a nano particle/nano-structured array, thermodynamics and kinetics must also be considered so a preferential binding can cause false readings. As in MALDI imaging, this issue must be solved, at which point SERS imaging could become a competing technique.
Duncan Graham
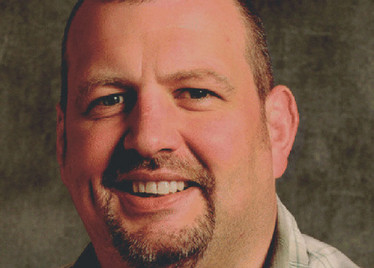
Duncan is Research Professor of Chemistry and Director of the Center for Molecular Nanometrology at the University of Strathclyde in Glasgow. He is currently Chair of the Editorial Board of Analyst and will serve in that role until 2018. He has been awarded numerous awards for his research including the RSCs SAC Silver medal (2004), Corday Morgan prize (2009), a Royal Society Wolfson Research Merit award (2010), the Craver Award from the Coblentz Society (2012), Fellows Award from the Society for Applied Spectroscopy (2012) and was elected to the fellowship of the Royal Society of Edinburgh (2008). He is a cofounder and director of Renishaw Diagnostics Ltd (2007) and has filed 15 patents with license deals on most of his portfolio. He completed a PhD in organic chemistry at the University of Edinburgh (1996) and his interests are in developing new diagnostic assays based on nanoparticles and spectroscopy with target molecules including DNA, RNA, proteins and small molecule biomarkers.
How do you see the role and impact of TERS (tip-enhanced Raman spectroscopy) expanding?
DG: I’ll leave this one to Volker!
VD: TERS is an interesting case – and my view on it cannot be neutral for obvious reasons. It is still experimentally demanding and most fundamental and application related research is found in academia. As it is one of the few techniques that allow us to address chemical composition with nanometer resolution, TERS is without alternatives in many cases. My expectation is that TERS will be tested on many different samples in the near future and either we will really learn about the desired nanoscale composition or equally important, learn about fundamental issues that prevent TERS detection in specific cases (diffusion, stability and so on). Clearly, TERS needs to move towards more general specimens with “normal” Raman scattering cross-sections, which will in turn have an impact on TERS mapping. In the near future my expectation is that most experiments will still be point measurements or profiles of specific areas of interest in combination with larger scale Raman and AFM images. Why? Because of fundamental physical laws in these dimensions. Positioning probes with sub-nanometer precision for a long time literally comes at a cost, but it could change dramatically when UV-TERS systems become available. Then an additional resonance Raman effect potentially decreases the measurement time such that imaging at a reasonable speed becomes feasible. In this regard, the instrument makers, in general, are heading in a very positive direction. And though their influence on the tip material and design has been low, the features of the instruments that allow a fast determination of potential areas of interest – whether Raman or AFM imaging – have improved a lot over the last few years. My expectation is that such developments will continue to help the development of novel TERS modes simply by focusing on the location of interest.
Considering the work done at EPFL, what do you think are the implications for analytical sciences?
VD: The EPFL approach is off the beaten track and provides a new route to the understanding of SERS. The challenge for all theoretical models for SERS is the intrinsic variability of the substrates. In this respect, most substrates for SERS were not produced using first principles, but rather in a trial and error fashion, which was, in some cases, extremely successful (for example, the proverbial Lee-Meisel colloid). Any directed method of SERS substrate manufacturing is of course very desirable and I am looking forward to seeing how this paper will affect practical SERS substrate design.
DG: The use of optical cavities could be very useful in SERS and it will be interesting to see the experimental SERS community building on this paper where some very exciting data is presented. However, SERS is a very complicated process and it does seem that the exact system being used or modeled can be unique, so it’s not always the case that data obtained or predicted in one SERS setup is transferable to others. The most obvious example of this is where several papers have shown a lack of dependence on matching the excitation frequency to that of the plasmon – but where as many papers show the opposite.
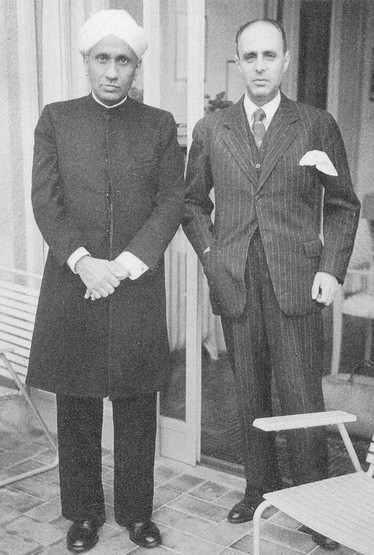
C. V. Raman with Swiss physicist (and banker) Richard Bär
How do you see the role of Raman developing in the near future?
DG: Raman has the opportunity to produce ultrasensitive analysis through some of the advanced surface enhanced or tip-enhanced Raman spectroscopy options. And because of instrumentation developments, low cost and portable Raman spectrometers are now available at a range of wavelengths, which opens up a range of new scenarios; for example, in vivo analysis, use outside laboratory environments, and use in the clinic at an acceptable cost. As noted, the key to the analytical performance of SERS lies in understanding and controlling the surface chemistry of any analyte species. This statement also applies to imaging techniques, where new microscope systems offer incredibly high performance when it comes to resolution and speed, but bring challenges in terms of data complexity, which demands the expertise of statisticians and the need for image processors. Raman and SERS imaging and mapping rival fluorescence in terms of the data; however, the techniques still acquire the images more slowly – but with the advantage that they can do so without labels. Ultimately, moving forward I suspect that a combination of fluorescence, Raman and SERS will start to be a necessary addition to the armory of the life or clinical scientist interested in studying single cells, tissues and complex biological systems.
VD: As indicated, I think the main impact of Raman will be in the field of biomedical analysis. Quick determination and identification of bacteria, viruses (potentially with TERS) will allow a faster response for infections. Compared to normal cultivation approaches, patients can gain up to several days. Similarly, for spectroscopic identification or supported identification of tumors during surgery, Raman is potentially very powerful. The challenges are similar to any other new treatment – trials take a long time and are very costly.
Normal Raman imaging is unlikely to compete with fluorescence-based techniques with respect to speed and lateral resolution. Raman imaging is competitive only if labeling is not possible or prohibitive. In terms of analytical challenges, we can also take from this that if little is known about a sample a-priori, Raman imaging is the best approach to get a first idea about a sample. Having said that, Raman can be more demanding than its competitors (IR, fluorescence) from an instrumental perspective. I will say that the stiff competition between the three has and will continue to be beneficial for them all. And I am pretty sure that none of these techniques will go out of business soon...
- P Roelli, C Galland, N Piro and TJ Kippenberg, “Molecular cavity optomechanics as a theory of plasmon-enhanced Raman scattering”, Nat Nanotechnol, 11, 164–169 (2016). DOI:10.1038/nnano.2015.264
- K Kneipp et al., “Single molecule detection using surface-enhanced Raman scattering (SERS)”, Phys Rev Lett, 78, 1667–1670 (1997).
- S Nie, SR Emory, “Probing single molecules and single nanoparticles by surface-enhanced Raman scattering”, Science, 275, 1102–1106 (1997). PMID: 9027306.
- DI Ellis et al., “Illuminating disease and enlightening biomedicine: Raman spectroscopy as a diagnostic tool”, Analyst, 138, 3871–3884 (2013). PMID: 23722248.
- X Qian et al, “In vivo tumor targeting and spectroscopic detection with surface-enhanced Raman nanoparticle tags”, Nat Biotechnol, 26, 83–90 (2008). PMID: 18157119.
- P Matousek et al., “Subsurface probing in diffusely scattering media using spatially offset Raman spectroscopy,” Appl Spectrosc, 59(4), 393–400 (2005). PMID: 15901323.
- D Graham et al., “Control of enhanced Raman scattering using a DNA-based assembly process of dye-coded nanoparticles,” Nat Nanotechnol, 3(9), 548–51 (2008). PMID: 18772916.
- S Ostovarpour et al., “Through-space transfer of chiral information mediated by a plasmonic nanomaterial”, Nat Chem, 7(7), 591–6 (2015). PMID: 26100808.
Volker Deckert holds a joint position at the Institute of Physical Chemistry at the University of Jena, Germany, and the Leibniz Institute of Photonic Technology, also in Jena. He obtained his Diploma and PhD from the University of Würzburg, Germany, working on difference-Raman spectroscopy. As a postdoc at the University of Tokyo and the Kanagawa Academy of Science in Kawasaki, he worked on non-linear and time-resolved laser spectroscopy of photo-induced isomerisation reactions. While at ETH Zurich, he started working on the development of high spatial resolution techniques for Raman spectroscopy, a topic that has followed him to Jena, where, in particular, he explores the possibilities of the technology to investigate structural changes of bio-related compounds with nanometer resolution.