Gurus of Single Molecule Detection
David Duffy, Ulf Landegren, Antoine van Oijen, and Menno Prins crash through the concentration barrier and investigate the ultimate limit of analytical sensitivity.
David Duffy, Ulf Landegren, Antoine van Oijen, and Menno Prins |
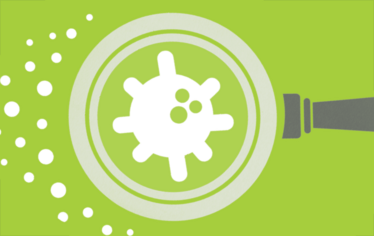
How are you involved in single molecule detection (SMD)?
Antoine van Oijen: My academic research group develops single molecule biophysical tools to study complex, multiprotein systems. We are interested in understanding the basic molecular mechanisms that underlie processes such as DNA replication, viral fusion, and membrane transport. As a professor and group leader, I focus on defining scientific projects, applying for funding, recruiting personnel (PhD students, postdoctoral fellows) and advising and mentoring them throughout their research.
Ulf Landegren: I am professor of molecular medicine at Uppsala University and I’m on the board of Olink Bioscience, a spinout company commercializing protein assays; I am also involved in a few other companies. Both in academic and industrial contexts, we are developing tools for life sciences; some of these are for single molecule level analyses for diagnostic applications.
David Duffy: We develop instruments for single molecule detection aimed at revolutionizing the measurement of proteins to improve healthcare. My role is developing and refining our fundamental single molecule array (Simoa) technology and associated instrumentation and I collaborate with clinicians and researchers to develop new applications.
Menno Prins: I am a professor at Eindhoven University of Technology and lead a research group that investigates molecular biosensing technologies, based on single particles and single molecules, for fundamental research and for near-patient testing applications.
Can you offer some historical perspective?
AvO: Up until a few decades ago, the ability to observe individual molecules seemed like science fiction. As a PhD student, I was fortunate to be involved in the early years of single-molecule detection, at a time when not many people worldwide were attempting to visualize single molecules in highly idealized model systems. Over the years, these tools have been applied increasingly to the understanding of how proteins work and have revolutionized how we think about molecular mechanisms and protein dynamics.
UL: Kary Mullis, inventor of PCR, saw how ridiculous it was to talk about 1.66 yoctomole instead of simply saying one molecule. Now, with digital PCR, we can count individual DNA molecules, and there are also routine detection techniques for single proteins, although not every single molecule is detected in these protein assays – it’s more like one out of every hundred or so.
SMD in life science applications requires great specificity as well as detection sensitivity to identify individual molecules in the vast complexity of real biological or clinical specimens. For example, our padlock probes can detect unique gene sequences or (reverse transcribed) transcripts. Once specifically reacted, the probes are amplified locally using rolling circle amplification (RCA) for convenient SMD by microscopy or in flow imaging.
Our proximity ligation assays (PLA) allow detection of individual proteins or protein complexes by pairs of antibodies modified with DNA strands (1). Upon proximal binding by the antibodies, the attached DNA strands guide the formation of DNA circles, which are replicated locally via RCA, just like padlock probes (2). This is now a standard single protein detection technique, combining specificity and sensitivity, which has more than 1000 users worldwide, and is applicable for analyzing clinical samples prepared for microscopy.
MP: The development of instrumentation for biochemical patient testing always starts with mechanical automation for increased throughput, and thereafter proceeds to a phase of miniaturization and integration to allow decentralized and more flexible testing. A sizable market has developed for decentralized point-of-care testing, with blood glucose testing being the most mature application. Point-of-care testing technologies continue to be developed for biomarkers such as small molecules, proteins, and nucleic acids. Beyond point-of-care testing, the next step in miniaturization and integration will be the development of devices for real-time patient monitoring, with biochemical sensors integrated into medical devices that remain for some time in contact with patients. This latter field is still in a very early phase. I believe that SMD technologies will progressively penetrate into all three segments: high throughput, point-of-care, and patient monitoring. In the single-molecule regime, every molecule counts and biology becomes digital, which can be advantageous for extracting maximum information out of a biological process.
DD: We developed a method for measuring proteins called “Digital ELISA”, a single molecule version of the analog enzyme-linked immunosorbent assay (ELISA) used for measuring proteins since the early 1970s. Our objective was to use single molecule sensitivity to drive huge increases in sensitivity over existing immunoassays. The technique has its roots in digital PCR, where single molecules are confined within very small volumes (picoliter to nanoliter) and quantification is achieved by counting them. To get digital ELISA to work for counting single proteins, you must measure single enzyme labels in wells that are much smaller (about 50 femtoliters [fL]) than those used for digital PCR. In 2005, David Walt at Tufts University and David Rissin, his graduate student, managed to do this with trapped solutions of beta-galactosidase in 50-fL well arrays etched into glass. The wells were sealed in the presence of the enzyme substrate with a silicone gasket. A single enzyme generates about 3,000 fluorphors in 30 seconds, and when sealed within 50-fL it can be detected using a standard laboratory fluorescence microscope. By counting enzymes in this way, we assumed that we could obtain huge increases in sensitivity to immune complexes labeled with beta-galactosidase. At Quanterix, we used this idea to develop digital ELISA by first capturing proteins on microscopic beads, labeling them with the enzyme, then sealing and counting them in femtoliter well arrays. We published this approach in Nature Biotechnology in 2010.
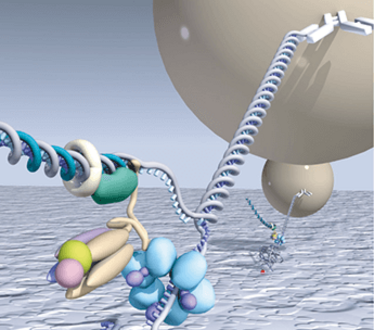
Artist’s impression of a single-molecule experiment that allows the visualization of the DNA-replication process at the single-molecule level.
A long DNA molecule is coupled between a bead and glass surface with a laminar flow mechanically stretching the tether. Tracking the positions of the individual beads reveals kinetic information on how fast the DNA gets unwound and duplicated by the protein machinery that supports replication.
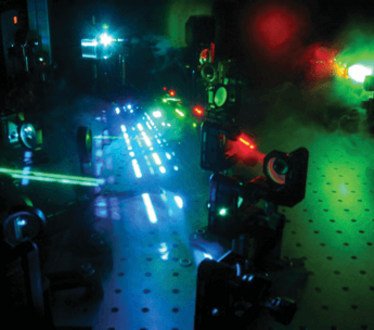
Fluorescence imaging tools have been developed that allow researchers to visualize the emission of individual, fluorescently tagged proteins. Lasers and optics are key components in these experimental approaches.
What is your view on current developments?
AvO: We are now at an exciting time when single-molecule biophysical approaches that unravelled basic molecular mechanisms are being used in biotechnological applications. The use of SMD in next-generation sequencing (Pacific BioSciences and Oxford Nanopores) and in super-resolution imaging (Nobel Prize Chemistry 2014) are the best examples. I think that many exciting developments will emerge in the future that will use single-molecule tools in diagnostic applications.
DD: SMD technologies are now entering the mainstream and are influencing the analytical measurements that scientists make. Digital PCR is now available in multiple platforms, and it is in wide use by researchers to study rare biological events. The improved quantitation of digital PCR is also helping adoption of this technology, and its implementation in diagnosis for humans is on the horizon. For our own protein detection technology, we are seeing widespread adoption from basic researchers in universities, to pharmaceutical companies developing drugs, to companies developing laboratory developed tests. By developing the technology for in vitro diagnostics, the dream of tests based on measuring single protein molecules will soon be a reality.
MP: Glucose testing represents the best example to date of near-patient medical biosensing. Rapid finger-prick blood tests are available, as well as devices for continuous glucose monitoring in skin. We should aim to measure much more than a patient’s glucose level, such as small molecules and proteins; however, these have much lower concentrations than glucose and it becomes very challenging to develop the necessary real-time monitoring technologies. In monitoring applications, sample preparation procedures should be minimized and direct detection is preferred. Current research focuses on miniaturized detection methods and novel molecular and cellular systems. The challenges are large, so new technological concepts are needed in this domain, not only for future applications in humans, but also for research on artificial tissues and other live biological systems.
UL: Single molecule analysis is increasingly in focus, including in the context of single cell analyses. There is a great demand for analyzing heterogeneity among cells and for precise and sensitive protein measurements. The protein assays we develop depend on simultaneous binding by sets of two, three or more antibodies for enhancing detection specificity and for single molecule sensitivity. The new capability of highly sensitive protein detection now provides opportunities – as well as a strong desire – to identify new generations of diagnostic protein biomarkers that depend on this increased sensitivity.
For you, what are the most important milestones?
UL: There has been much progress in miniaturizing SMD of proteins, which confines reaction products to volumes where nonspecific background species are negligible. By contrast, we have solved SMD problems at both nucleic acid and protein levels by establishing new probe designs that depend on recognition of two or more affinity reactions before amplified detection. The design of the assays means that only the correct combination of reagents can give rise to detectable signals, thus enabling multiplexing without associated background problems - another important aim of current molecular assays.
DD: First, the demonstration of a thousand-fold improvement over standard ELISA that we published in Nature Biotechnology (3). Second, the low cost consumable that we developed with Sony DADC, which showed that we could provide cost effective single molecule analysis, making it accessible by the wider biomedical community. Third, the commercial launch of our fully automated instrument that gave researchers access to the sensitivity improvements provided by Simoa. In terms of clinical milestones, we demonstrated a test that detected cancer recurrence months rather than years after surgery; we showed that protein biomarkers of neurological disorders could be measured directly in blood; and we showed that Simoa could detect viruses and bacteria at the same levels as nucleic acid testing.
AvO: I think one of the biggest milestones was psychological: the technology for detecting the fluorescence of a single molecule has been around since the 1970s (lasers, sensitive photodetectors), but it had been considered impossible to detect the weak fluorescence of a single molecule amidst background contributions. Throughout the 1990s, and with the first demonstrations of SMD, it became clear that the technical challenge was not necessarily in the physical detection equipment, but rather in the selective and specific labeling of biomolecules needed to allow single-molecule biophysical studies without perturbing function.
MP: In the coming years, point-of-care biosensors will come to market for small-molecule and protein testing with an analytical performance level that meets laboratory equipment. In the long term, I think an important leap will be the development of technologies for continuous biochemical monitoring of patients, integrated into medical devices and disposables.
How does SMD fit into the broad picture of analytical science?
AvO: Strictly speaking, single-molecule studies represent the ultimate analytical science in that the focus is on the development of tools to analyze biological processes at the ultimate sensitivity level.
DD: Our founder and early employees were analytical chemists, and we are dedicated to developing analytical methods based on SMD. Measuring very low concentrations is also in our genes. It is one thing to detect if a molecule or organism is present in a sample, but having the ability to precisely and accurately measure the concentration of molecules across a wide dynamic range is a large technological challenge, albeit a somewhat unglamorous one. It’s something we strive to do well even when making multiplex measurements a thousand times lower in concentration than normal!
UL: Obviously, there is a desire to measure rapidly increasing numbers of biomolecular properties in the human body, both for healthcare and in wellness research. There is also a rapid price reduction per datapoint, balanced by vast increases in amount of data collected. One particular instance of single or rare molecule detection that will increase in importance with the advent of improved tools is measuring exceedingly rare mutant versions of genes present in blood plasma from tumor patients for monitoring minimal residual disease.
MP: Our research includes the development of detailed mechanistic understanding of interactions between molecular probes, particles, surfaces, and complex biological matrices. Specific and non-specific interactions, multivalency, protein coronas, heterogeneities in space and time, for example. Research methodologies based on single-molecule resolution will generate breakthrough insights into these complex questions that are very important for analytical science.
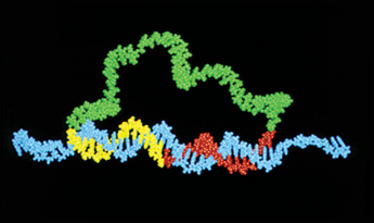
Padlock probe for single molecule DNA detection. Single molecule detection in complex biological samples requires excellent specificity of recognition, and detection signals that exceed any background. Padlock probes achieve single molecule detection by depending on target recognition by the two ends of the probe (yellow and red). This allows the ends of the probes to be joined by DNA ligation, giving rise to a circular DNA strand, which can be locally amplified by a process of rolling circle amplification for easy visibility.
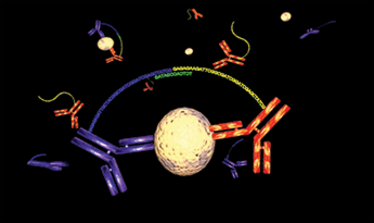
Proximity ligation for single protein molecule detection. Pairs of antibodies specific for a protein of interest are conjugated to DNA strands. When both antibodies bind a target protein the DNA strands are brought in proximity and can be joined by DNA ligation. Ligated DNA strands can then be amplified for easy detection using molecular genetic techniques such as PCR or rolling circle amplification. Illustration by Jonas Jarvius.
What is your mission for society, health and science?
DD: We wish to inspire a revolution in personalized healthcare by exploiting the power of the protein. Most of the incredible progress in molecular diagnostics and personalized medicine in the last 10 years or so has been driven by detection of nucleic acids. This revolution is due to two major analytical developments: nucleic amplification technologies (like PCR) and next-generation sequencing. We believe, however, that protein is a much richer, biologically-relevant molecule for detecting disease, so has a greater potential for understanding biology and improving diagnosis. Simoa is a major development that I believe will spark a revolution in protein-based molecular diagnostics in the next few years. Other technologies will also contribute to this coming revolution, including next-generation mass spectrometry techniques that can drive the discovery of new protein markers, and new approaches to protein-binding reagents that allow these new markers to become validated diagnostics.
UL: Our aim is to improve human health, both in the developed and developing countries around the world, primarily by permitting earlier diagnoses in the course of disease, in preference to late and chronic therapy. Although we work in academia, successful technologies are transferred to industry to make the methods more broadly available.
AvO: The mission of our academic group focuses on understanding molecular mechanisms. Our main goal is to develop single-molecule technology that allows us to understand how DNA is replicated. While this goal is driven by basic science, the technological spin-offs have an impact in diagnostic tools, and the results of using these tools contribute to greater understanding of disease mechanisms and antibiotic resistance.
MP: My mission is to train and inspire young scientists and engineers by involving them in top-level biosensing research. We investigate and develop novel biosensing principles based on single particles and single molecules, for fundamental research as well as for applications in healthcare. An important aim in healthcare is to enable people to live a high-quality life in their own environment. Novel biosensing technologies will help to achieve this aim, by recording the biochemical health status of people in need of care.
What are the major hurdles?
MP: Science involves new ideas, consistent working – and of course some luck. For biosensing research, good collaborations are needed between experts in physical sciences and molecular engineering. And finally, special skills are required for the translation of scientific results to medical applications, which is the topic of YouTube video “Translate to Innovate” that I have recently published (4).
UL: It takes a long time to develop a molecular tool and a successful technique, so that they can be widely available for parallel, highly sensitive molecular analysis. It can’t be rushed; and, in our case this process regularly extends over more than 10 years. Most of the delay is in finding the right student or students for the job, but we have been blessed with many talented students.
DD: The first hurdle was time. As a small company with a big idea, we needed the time and money to bring the idea to fruition. Fortunately, we had understanding investors who funded the early development of the technology. We were also fortunate to receive support from government agencies and collaborators. The second hurdle was scalability. A big idea can only become really big if it can meet the financial and implementation expectations of customers who have high demands for both – again a challenge for a small company. And that’s why we partnered with some larger companies to achieve our vision.
AvO: While our research group receives the funding we need to pursue our scientific goals, I am worried about the reduction in funding for basic sciences and the shift of focus to translational efforts. In the long term, success as a society in research and development needs both types of research. Shifting the balance to applied work may result in economic benefits in the short turn, but we shall lose momentum in the end.
Tell us about some of your successes.
DD: Publishing in Nature Biotechnology demonstrated to the world that we had a scientifically-sound approach to SMD. It created a lot of credibility and interest for the technology. Launching a fully automated single molecule instrument just two years after development began was also a significant achievement, and we now have dozens of customers using the instrument around the world. It’s just the start for Simoa, but it is exciting to see all the amazing uses of SMD.
UL: It is a great pleasure to see our methods in widespread use, even if not always correctly attributed. Some eleven large biotech or diagnostic companies have licensed techniques from our lab, and we are now spinning out our sixth company.
AvO: I feel fortunate in being able to work with a fantastic group of researchers who are all driven by a desire to understand the world around us and to leverage that understanding for the betterment of society.
MP: While working at Philips Research, I initiated and then developed with a team a point-of-care biosensing platform based on magnetic nanoparticles (5, 6). In this technology, all assay steps are controlled by electromagnetic forces, and for low biomarker concentrations the nanoparticles are bound to the sensing surface by single molecular bonds. I became a fulltime professor at Eindhoven University of Technology last year, and my aim is to once again develop innovative biosensing technologies, starting from novel basic concepts.
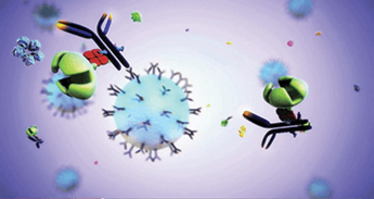
Single protein molecules are captured and labeled on antibody-coated beads.
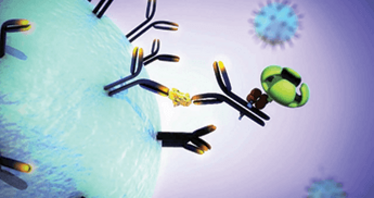
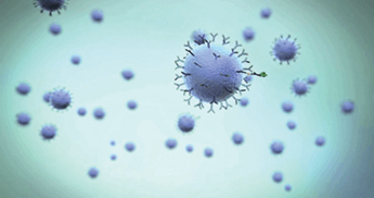
Single beads are isolated in arrays of 216,000 50-femtoliter wells.
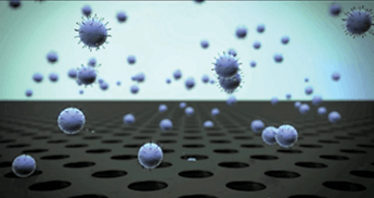
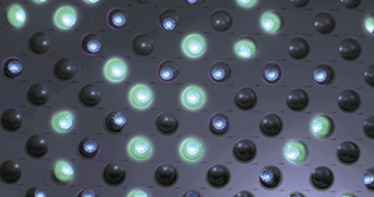
Single molecules are subjected to fluorescence imaging.
How do you hope your work impact society?
AvO: We want to understand in molecular detail how the bacterial DNA-replication machinery works. By combining our single-molecule biophysics efforts with modern structural biology, biochemistry, and computational modeling, I feel we should be able to make great progress over the next three to six years. Such knowledge will help us with understanding antibiotic resistance and potentially identifying new approaches that will help us with this problem.
UL: In the following order: I am hoping that we will have identified new analytical challenges, proven that those we are currently working on are robust, and brought more of the earlier ones to clinical applications. Simple!
MP: My goal is to address the question of how single-particle and single-molecule methodologies can improve biosensing. And, in the long term I hope to contribute to a new sensing technology that is suited for integration into medical tools and for real-time biochemical monitoring of patients.
DD: We hope to be well on our way to achieving our mission of changing healthcare through measuring proteins. In three to six years, the Simoa technology should be widely adopted in the research market and being used for in vitro diagnostics. I am confident that many new insights and applications for measuring single protein molecules will have emerged in that time. We also hope to have expanded the use of the technology into new markets, like point-of care, and to have augmented Simoa with other enabling protein technologies. Hopefully, a protein molecular diagnostics revolution will be well underway!
- O. Söderberg et al., “Direct Observation of Individual Endogenous Protein Complexes in situ by Proximity Ligation”, Nature Methods 3, 995-1000 (2006).
- M. Nilsson et al., “Padlock Probes: Circularizing Oligonucleotides for Localized DNA Detection”, Science 265, 2085-2088 (1994).
- D. M. Rissin et al., “Single-Molecule Enzyme-Linked Immunosorbent Assay Detects Serum Proteins at Subfemtomolar Concentrations”, Nat. Biotechnol. 28, 595–599 (2010).
- M. W. J. Prins, “Translate to Innovate”, tas.txp.to/0515/translate (2015).
- D. M. Bruls et al., “Rapid Integrated Biosensor for Multiplexed Immunoassays Based on Actuated Magnetic Nanoparticles”, Lab Chip 9, 3504-3510 (2009).
- A. van Reenen et al., “Integrated Lab-on-chip Biosensing Systems Based on Magnetic Particle Actuation – a Comprehensive Review”, Lab Chip 14, 1966-86 (2014).