Life As We Don’t Know It
SHERLOC – together with its camera sidekick WATSON – will use Raman and fluorescence spectroscopy to seek out the molecular signatures of life on the Mars 2020 mission.
Luther Beegle |
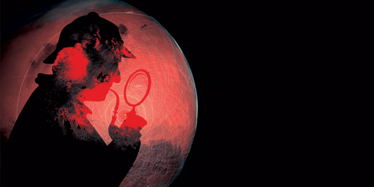
The Scanning Habitable Environments with Raman and Luminescence for Organics and Chemicals (SHERLOC) instrument will be mounted on the robotic arm of the Mars 2020 rover. SHERLOC enables non-contact, spatially resolved, and highly sensitivity detection and characterization of organics and minerals on the Martian surface and near subsurface, with four main goals:
- To assess the habitability potential of a sample and its aqueous history
- To detect the availability of key elements and energy sources for life (for example, carbon, hydrogen, nitrogen and many others)
- To determine if there are potential biosignatures preserved in Martian rocks and outcrops
- To provide organic and mineral analysis for selective sample caching.
SHERLOC is a resonance Raman and fluorescence spectrometer with a 248.6-nm deep UV laser. Deep UV-induced native fluorescence is very sensitive to condensed carbon and aromatic organics, enabling detection at or below 1 ppm at 100 µm spatial scales, while deep UV resonance Raman enables detection and classification of aromatic and aliphatic organics with sensitivities of 0.01 to 1 percent at 100 µm spatial scales. In addition to organics, the deep UV Raman enables detection and classification of minerals relevant to aqueous chemistry with grain sizes on the order of 20 µm. SHERLOC will be able to map the distribution of organic material against the visible features and minerals that are identifiable with the Raman spectrometer.
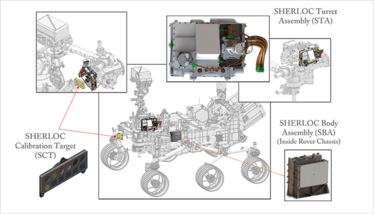
It’s important to understand that SHERLOC is not a life detection instrument in the classic sense of the word “life.” We do not believe a single instrument could find life on Mars, for two reasons: first, there is so much uncertainty as to what life on Mars might look like that it’s hard to imagine a single instrument unambiguously identifying it; second, given what we know of present day Mars, if life “as we know it” were present, it would be very scarce indeed – below the limits of detection of any instrument.
Sowing the seeds
In 1996, a research paper claimed to have found life in a Martian meteorite called ALH84001 (1). The debates that followed amongst planetary scientists and astrobiologists – while ultimately concluding that the meteorite did not contain life – highlighted that we had little clue how to look for life on another planet, or even what to look for.
Intrigued by the problem, the team here at NASA’s Jet Propulsion Laboratory (JPL) embarked on a series of projects developing instruments capable of identifying life. SHERLOC came out of that effort. Ten years ago, my colleague Rohit Bhartia was leading a Raman spectroscopy-based project, while I was working on mass spectrometry-based instrumentation – both with the aim of detecting “life” on Mars. By 2008, Rohit and I were talking on an almost daily basis to compare our different strategies, and identify what we would be looking for. We both agreed early on that to definitively identify life, you need multiple instruments that make multiple different types of measurement.
At around the same time that ALH84001 ignited a debate about life on Mars, there was a revolution in how we think about life here on Earth. Microbial life was found in an array of seemingly unlikely places – several kilometers underground, in the dry valleys of Antarctica, in saline lakes, and in highly alkaline environments. It proved that life can exist in the most inhospitable places, and Rohit and I talked a lot about how we would find life in those harsh environments, and how that might translate to finding life on Mars.
In 2013, when the Mars 2020 Science Definition Team (who are responsible for defining the objectives and capabilities of the mission) announced that instruments would need to interrogate at the 100 micron spatial scale, with minimal sample handling, we ruled out mass spectrometry and turned all our efforts towards Rohit’s spectroscopy-based concept.
Birth of a master detective
We spent the first part of 2013 putting together the concept so that it could be ready for when the instrument Announcement of Opportunity was released that summer. It helped that we could develop the concept with an idea on how things worked on the Mars Science Laboratory (MSL) mission, which landed in August 2012. For example, we knew that the robotic arm had inaccuracies in placement of up to a cm, so an autofocus mechanism would be key; we talked to the developer of the Mars Hand Lens Imager (MAHLI) on the MSL and figured out how to incorporate their hardware. By leveraging existing hardware, we could concentrate on developing the “higher risk” items, such as the laser.
We went through a lot of internal JPL reviews, and naturally everyone had an opinion on what we should or should not include, with plenty of contradictory advice – all of which we listened to (and some of which we followed!). We had an excellent team to help us put the concept together, including an awesome system engineer, Lauren DeFlores, who made sure that everything fit together into a neat little package.
In July 2014, I got the call to say we’d been selected for inclusion in Mars 2020, while on vacation with my family in Breckenridge. It was lucky that I was away from JPL, or I’m not sure I could have survived the 24-hour embargo on sharing the good news.
A look inside
Our main challenges come from Mars itself – an inhospitable place for scientific instruments. It is an optical instrument attached to a vibration-inducing coring mechanism at the end of a robotic arm that experiences 100 ˚C temperature variations on a daily basis – in a very dusty environment. Plus, we have to operate with low power and limited data volumes.
There are two optical assemblies in SHERLOC (see Figure 1), the one on the right is called WATSON, and is a reflight of the MAHLI, which is currently on MSL. WATSON is a variable focus color imager that can take microscopic images of science targets as well as images for engineering purposes, such as recording wear on the robotic wheels. The Autofocus Contextual Imager (ACI) on the left allows us to obtain a black and white image of a surface, as well as autofocusing so we can do laser spectroscopy. The laser spectroscopy takes place at a working distance of 48 mm from a surface, plus or minus 7 mm
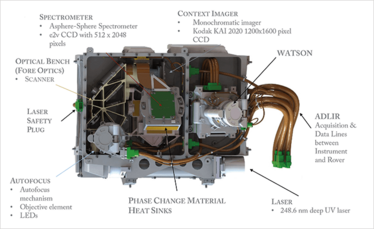
Figure 1. Major SHERLOC assemblies.
There are two other pieces of hardware – an electronics box inside the rover and a calibration target. The calibration target allows us to verify the instrument’s performance on the surface of Mars and includes a piece of a Martian meteorite and several pieces of spacesuit material of a type that would be used when humans eventually make it to Mars; by exposing the material to the environment of Mars, we can assess if it degrades with time.
SHERLOC is one of seven instruments being developed for Mars 2020. We share the arm with the PIXL instrument, which produces elemental maps of surface material. There are two instruments on the mast, MastCam-Z and SuperCam. MastCam-Z takes color stereo images of the surface, and SuperCam has the ability to take Raman spectra at a distance of up to a few meters, laser induced break down spectroscopy (LIBS) from up to 10 meters and IR spectra from even further. All these measurements will help us understand the geologic context of a sample, over multiple spatial scales.
In this mission there is very little ability to do sample handling, because the caching system is so complex. On MSL, samples can be obtained through a rotary percussive drill that pulverizes a sample, feeds it through a sieve and then into a measuring tube, before it is delivered to the Sample Analysis at Mars (SAM) instrument. It was not feasible to incorporate all that hardware on Mars2020. Plus, Mars has a significant amount of perchlorate on the surface – on heating, the perchlorate destroys organics, meaning that conventional GC-MS destroys the very molecules that you are looking for. SHERLOC is a very low power instrument, and we can see organics and perchlorates without causing a chemical reaction.
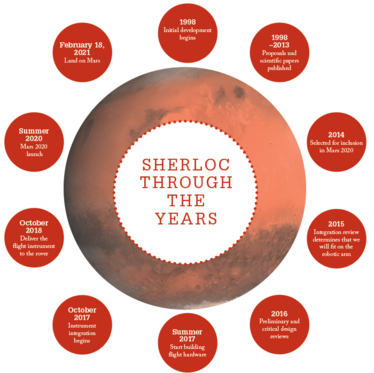
What are we looking for?
The two different types of spectroscopy in SHERLOC have different but complimentary uses. Fluorescence spectroscopy (with a 248 nm laser) detects poly aromatic hydrocarbons (PAHs) – a very strong signal that allows for sub-ppm detection; the fluorescence signature starts at around 273 nm and continues into the visible.
Raman spectroscopy allows us to identify aliphatic organic material as well as study mineralogy. The Raman region starts at 252 –273 nm and the signal is up to six orders of magnitude weaker than fluorescence. The use of the 248.6 nm laser allows us to get the Raman and fluorescence on the same charge-coupled device (CCD), thereby doubling the amount of information we obtain with each laser pulse.
We will look for aromatic organics using fluorescence and aliphatic organics using Raman spectroscopy. We will also search for minerals that are astrobiologically relevant because they were created in aqueous environments, such as carbonates, phyllosilicates, and so on.
Potential biosignatures could include:
- A significant amount of organics in conjunction with minerals that formed in aqueous environments
- A significant deposit of aliphatic organics right under the surface
- Layers of organic molecules that correspond to specific elements that PIXL quantifies.
It is a real challenge to tell the SHERLOC story without generating sensational headlines about “life on Mars”. We are able to detect organic molecules in very trace amounts in very small areas, and this will inevitably cause some people to think we will have found life. Even today, there are a handful of researchers who believe that they found life on Mars in the 1970s, during the Viking mission, while others insist that ALH84001 shows genuine signs of life. While we are excited about the possibility of what we will find, we believe that extraordinary claims require extraordinary evidence and that definitive proof of life requires multiple lines of evidence from multiple instruments.
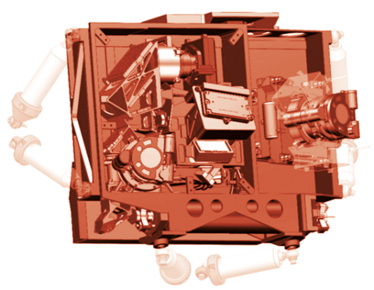
Mission status
Currently, we have around 30 full-time employees, including our project managers Ed Miller and Randy Pollock. The team consists of system engineers, and cognizant engineers for subsystems, such as optics, electronics, data handling and so on – as well as integration and test teams who actually build the instrument. We also work with a number of external companies and agencies (see “Mission Namecheck” below).
Right now, our major challenges are twofold: firstly, getting each subsystem to operate under all the conditions that we will encounter for two Martian years (three Earth years); and secondly, to make sure that all the hardware from each vendor and subsystem comes together at the right time so that it can be integrated together. We have a very compressed period of integration and if a part is delivered late, it can really affect other subsystems, no matter how small that part is. We deliver the instrument for integration into the rover on October 31, 2018. Until then, we are building, assembling and testing the instrument to demonstrate that it will survive the planet’s harsh environment.
Mission Namecheck
SHERLOC has three charge-coupled devices (CCD) and three optical paths, along with multiple power supplies, two separate computers, two moving parts and multiple electronics assemblies. It’s a huge task and includes the work of five subcontractors:
- Malin Space Sciences: ACI and WATSON hardware
- Los Alamos National Laboratory: CCD, command and data handling
- Left Hand Design Corporation (LHDC): scanner mirror
- Photon Systems: laser
- Johnson Space Center: calibration target
We started developing the concept in 1998 and SHERLOC itself in 2013. When it lands on February 18, 2021, we will have been working on it for seven years. It has been a very exciting (and stressful) four years so far and we now have less than a year left before we deliver the flight instrument to the rover. There is a JPL video called “Seven Minutes of Terror” that describes entry, decent and landing for MSL (https://www.youtube.com/watch?v=Ki_Af_o9Q9s), and we like to joke that this project is putting us through our own “Seven Years of Terror”! (My coping strategy is running – three years ago, I could barely run a mile, and in November 2017 I finished my first marathon!) For now, SHERLOC takes up about 20–30 percent of my time, but that will rise to 100 percent by the time we land and for the first few years on Mars.
All our families share our excitement (and nerves). During the MSL landing, my 10-year-old son held my hand tighter than I could have imagined, because he knew that I had put four years of hard work into this project, and that if something went wrong it would be the loss of my dream. My son is now 6 feet 4 inches tall; by the Mars 2020 landing he will be a college student – and I hope he will hold my hand just as tightly.
Acknowledgement
The research was carried out at the Jet Propulsion Laboratory, California Institute of Technology, under a contract with the National Aeronautics and Space Administration.
- McKay et al., “Search for past life on Mars: possible relic biogenic activity in Martian meteorite ALH84001”, Science, 273, 924–930 (1996).
Principal Investigator, SHERLOC, and Deputy Division Manager Science, NASA Jet Propulsion Laboratory, California Institute of Technology, Pasadena, California, USA.