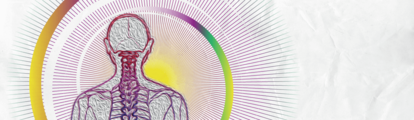
Operation Spectroscopy
In the quest to improve patient outcomes, collaborations between surgeons and spectroscopists are leading the charge with real-time measurements that could take some of the guesswork out of surgery
A recurring theme in the analytical sciences is the movement of techniques out of the lab and into the field. In past issues of The Analytical Scientist, we have put the spotlight on spectroscopic guns to extract information from ancient art, portable GC-MS systems for the rapid identification of hazardous chemicals at fire scenes, and portable analyzers to ensure quality of consumer products at all phases of production. But perhaps the most exciting stage of all is the operating theater.
Already, patients going under the knife may come under the scrutiny of advanced analytical instruments – all in the name of improved treatment and outcomes. These efforts are visible in success stories like the iKnife (a surgical instrument that identifies cancerous cells by rapid evaporative ionization MS intraoperatively) and MasSpec Pen (a similar tool using ambient ionization MS). Arguably less publicized, however, is the expanding role of spectroscopic approaches in this space.
Do spectroscopic approaches have the potential to change the way we operate? In what areas will they make the biggest impact? And what will these changes mean for the way analysts and clinicians collaborate? We spoke to two specialists – one surgeon and one spectroscopist – to answer some of the key questions.
Meet Our Contributors
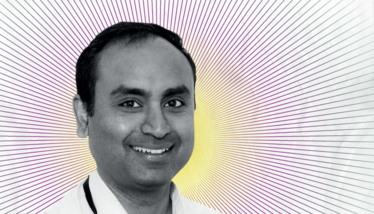
The Surgeon: Saba Balasubramanian
“My interests lie in the surgical management of primary hyperparathyroidism and thyroid disease – using novel technologies and evidence-based approaches to achieve the best possible outcomes for patients. I am an Honorary Professor at the University of Sheffield, and have been conducting this work since 2010. I have also acted as Principal Investigator on a number of animal and clinical studies in this space, and am currently Chief Investigator on a project exploring the clinical utility of near-infrared fluorescent imaging in thyroid surgery. The aim? To enable the real-time differentiation of the thyroid and parathyroid glands and reduce human error in the operating theatre.”
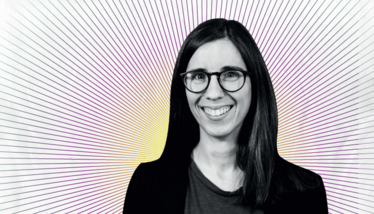
The Spectroscopist: Alba Alfonso-García
“When training as a physicist, I soon realized that a number of the tools developed in physics and chemistry labs could be applied by biologists and doctors. That’s when I decided to join Laura Marcu’s team, drawn in by her unique expertise in translating imaging tools into the clinic. Now I work as a Project Scientist at the University of California, Davis, developing optical imaging tools for biomedical applications. My team uses fluorescence lifetime imaging for disease diagnosis, including the detection of tumor margins during neurosurgery.”
Spectroscopy at the cutting edge
Spectroscopy isn’t new to the clinic. Even well-established practices like cervical cancer screening may utilize electrical impedance spectroscopy (EIS) to differentiate between healthy, premalignant and malignant tissues based on their respective resistance and capacitance properties. And we are seeing spectroscopy increasingly applied in more challenging clinical scenarios, including surgical procedures.
“Spectroscopic techniques have been used in intraoperative contexts for a number of years now,” says Alba Alfonso-García (Project Scientist in Photonics at the University of California, Davis). “Fluorescence lifetime imaging (FLIm), Raman, near-infrared (NIRS) and diffuse reflectance spectroscopy – as well as many others – have all been utilized for such purposes.”
The applications for spectroscopic analyses during surgery are varied. For example, studies have shown a benefit for perioperative NIRS monitoring of the tissue oxygenation index – which predicts the occurrence of ischemic intolerance and cerebral hyperperfusion (adverse events with significant patient morbidity) – during carotid artery stenting (1). NIRS has also shown potential as a predictor for shunt requirements during carotid endarterectomy by tracking cerebral oxygenation to predict cerebral ischemia (defined by insufficient blood flow to the brain) (2). And it’s not just human surgeons who benefit. Robot-assisted surgery – in which spectroscopic approaches represent a potentially powerful tool for defining target tissues – is becoming increasingly widespread (Figure 1) (3).
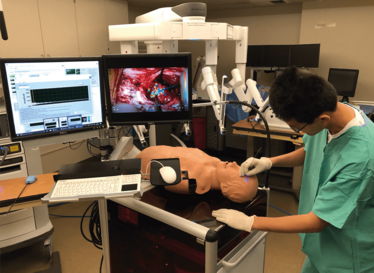
Figure 1. Graduate student Tianchen Sun (Marcu Laboratory, UC Davis) testing the FLIm instrument on the training suit of the UC Davis Medical Center
In oncology, promising results in both animal models and excised tissues (alongside key instrumental advances) has allowed studies to progress into the clinic, with a number of human trials underway (4,5). But, as Saba Balasubramanian (Honorary Professor in Surgical Oncology at the University of Sheffield) explains: “Spectroscopic approaches are currently applied only in early-phase clinical trials in oncology, and remain relatively novel in the operating theatre.”
Despite numerous challenges, both Alfonso-García and Balasubramanian are determined to continue evaluating the utility of spectroscopic techniques as a regular part of the surgical toolkit.
A guiding light?
Alfonso-García’s research focuses on identifying and highlighting tumor tissue during resection surgery. “Being able to identify tumors in situ – without the need to treat patients with external labels – means that spectroscopic techniques are minimally invasive and thus very promising,” she says.
Her group, led by Laura Marcu, has developed a number of approaches that use fluorescence lifetime spectroscopy to diagnose and guide the removal of tumors. The rationale? To remove the subjectivity involved in resecting tissue on the basis of visual inspection during surgery. While the current gold-standard imaging approaches (magnetic resonance imaging and positron emission tomography-computerized tomography, for instance) are helpful in guiding tumor resection surgeries, they are carried out pre-surgery and cannot highlight potential tissue changes caused by drift or swelling during procedures, explains Alfonso-García.
Balasubramanian echoes the need for real-time information. “The current approach for differentiating the parathyroid glands (each of which is approximately the size of a grain of rice) from normal thyroid tissue relies largely on surgeon experience and judgment – more objective approaches are needed,” he says. Inadvertent damage to parathyroid glands during thyroid surgery can lead to post-surgical hypoparathyroidism – a condition associated with a variety of distressing symptoms, ranging from muscle cramps to seizures and cardiac disturbances, that can impact on patients’ quality of life.
In order to reduce such errors, Balasubramanian and colleagues applied EIS to differentiate between thyroid and parathyroid tissues in 56 patients in a Phase I clinical study – the first of its kind (6). The department’s previous work using rabbit models had provided proof of principle, but as with all first-in-human trials, there was no guarantee the animal findings would translate successfully to humans. To the team’s delight, the technology – which used a handheld device with disposable, single-use sensor covers comprising four gold electrodes arranged in a square across a diameter of 5.5 mm – demonstrated good sensitivity and specificity when handled by two consultant endocrine surgeons.
Alfonso-García’s team has enjoyed similar success, in this case using FLIm instruments integrated with surgical microscopes to provide real-time modification of the surgical field of view and provide diagnostic information to surgeons (Figure 2) (7). “This provides surgeons with an extra dimension of information based on tissue structural organization and metabolism, in addition to the preoperative information and visual cues already available to them,” says Alfonso-García. “The main benefit for patients is that these measurements are innocuous – the probe doesn’t touch the body and there is no discomfort involved. Other imaging techniques, by contrast, tend to use ingested or injected contrast agents or ionizing radiation.” But it’s the ability for surgeons to respond to real-time data and so improve surgical outcomes that will drive these approaches out of the laboratory and into the clinic.
Other successes from the UCD lab include real-time guidance during robotic oral cancer surgery, assessment of radiation-induced necrotic changes in the brain, and automated breast cancer detection in resected specimens.
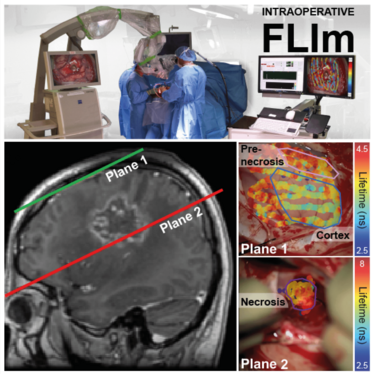
Figure 2. Graphical abstract showing an intraoperative fluorescence lifetime imaging instrument used to resolve distinct tissue types and pathologies including cortex, tumors, and radiation-induced necrosis during human brain surgery; the instrument integrates with surgical microscopes to provide real-time intraoperative augmentation of the surgical field of view, complementing information from pre-operative MRI
Spectroscopy and surgery: a match made in heaven?
The research is certainly promising - but how close are we to seeing these techniques in everyday use by surgeons? Speaking of his success in the application of EIS to (para)thyroid differentiation, Balasubramanian was quick to highlight the limited spread of these techniques at present. “Right now, these methods are being used only in clinical trials,” he says. “As we know, these studies are often limited to the ivory towers or Centers of Excellence.” Indeed, Alfonso-García highlights that her FLIm method to guide neurosurgeons is currently only conducted at the high-tech University of California Medical Center in Sacramento. The team is working on cloning the device and establishing partnerships with more universities and institutes. But, for now, it remains – in Alfonso-García’s words – “a clinical research approach.”
The first step towards moving either of these approaches into regular clinical practice will be to demonstrate utility in a wider cohort of patients. For Alfonso-García’s team, this effort is characterized by identifying numerous cases in which the approach is effective and subsequently applying advanced data analysis techniques to classify future measurements as healthy versus not. In the case of the acquisition of very large amounts of data, even more nuanced distinctions between tissue types or degrees of malignancy may even be possible. Movement into additional therapy areas such as head and neck and breast cancers is also currently underway.
“Improvements in augmented and mixed reality hardware (in the form of headsets that augment the visible tissue landscape or a modified display on a screen) will also be of benefit to advance these approaches and make them more user-friendly,” says Alfonso-García. “In addition, full-field systems (three-dimensional measurement systems that capture the entire field of view) may support certain applications by providing a complementary method to the 'painting' approach that we currently have, based on point measurements. Similarly, advances in data analysis techniques (including machine learning and other artificial intelligence approaches) will provide more refined and sophisticated data interpretation and visualization schemes.”
Balasubramanian’s team is concentrating on the more traditional route to the clinic. Following their successful Phase I trial in fluorescent imaging, they have secured funding to proceed to Phase II and III trials. With regards to their electrical impedance device, a number of changes are required to optimize the instrument for use in the operating room. Namely, the surface area of the sensor tip will need to be reduced and measurement acquisition will be modified to occur in real-time.
The other necessary ingredient to bring these approaches into routine use? Collaboration, according to our contributors. As in all areas of scientific research, well-organized collaborative efforts will lead to faster progress, says Balasubramanian: “Do we identify a clinical problem and then try to identify analytical techniques to solve it, or do we begin with the technology and look for clinical applications? Cross-disciplinary teams are needed to bridge these two approaches and lead the way in the field.”
- Terakado et al., “Effectiveness of near-infrared spectroscopy (NIRO-200NX, Pulse Mode) for risk management in carotid artery stenting”, World Neurosurg, 131, e425 (2019). DOI: 10.1016/j.wneu.2019.07.184
- Jonsson M et al., “Near infrared spectroscopy as a predictor for shunt requirement during carotid endarterectomy”, Eur J Vasc Endovasc Surg, 53, 783 (2017). DOI: 10.1016/j.ejvs.2017.02.033
- Gorpas D et al., “Autofluorescence lifetime augmented reality as a means for real-time robotic surgery guidance in human patients”, Sci Rep, 9, 1187 (2019). DOI: 10.1038/s41598-018-37237-8
- Livermore LJ et al., “Rapid intraoperative molecular genetic classification of gliomas using Raman spectroscopy”, Neurooncol Adv, 1, vdz008 (2019). DOI: 10.1093/noajnl/vdz008
- Eissa A et al., “’Real-time’ assessment of surgical margins during radical prostatectomy: state-of-the-art”, Clin Genitourin Cancer, S1558 (2019). DOI: 10.1016/j.clgc.2019.07.012
- Hillary SL et al., “Use of electrical impedance spectroscopy for intraoperative tissue differentiation during thyroid and parathyroid surgery”, World J Surg, 44, 479 (2020). DOI: 10.1007/s00268-019-05169-7
- Alfonso-Garcia A et al., “Real-time augmented reality for delineation of surgical margins during neurosurgery using autofluorescence lifetime contrast”, J Biophotonics, 13, e201900108 (2020). DOI: 10.1002/jbio.201900108
I've always wanted a job that fosters creativity - even when I worked on the assembly line in a fish factory. Outside work, I satisfy this need by writing questionable fiction. The venture into science writing was an unexpected departure from this fiction, but I'm truly grateful for the opportunity to combine my creative side with my scientific mind as Editor of The Analytical Scientist.