Researchers from the University of Massachusetts Amherst, USA, have designed a cost-effective device to identify per- and polyfluoroalkyl substances (PFAS) – the group of “forever chemicals” that resist environmental degradation and are associated with a wide range of health complications.
Using biological nanopores with a cyclodextrin adapter, the device is capable of identifying PFAS levels as low as 400 parts-per-trillion (ppt).
We reached out to co-authors Chang Liu and Xiaojun Wei to find out more about how the instrument was developed, and to get their perspectives on how the approach compares to current methods.
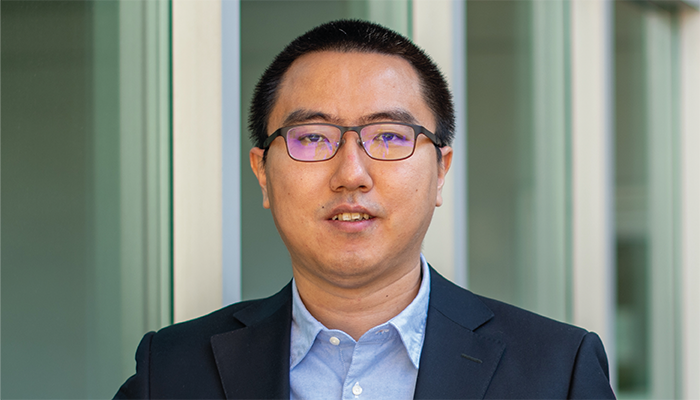
Chang Liu
Credit: Supplied by Interviewee
Could you describe how your device works?
We utilized single-molecule nanopore technology as a promising approach for developing portable detection devices, specifically employing α-hemolysin (α-HL) nanopores embedded in a liposome membrane to create a single-molecule channel. This setup enables the characterization of molecules within the pore by detecting changes in ionic current signals. To target PFAS molecules, we used γ-cyclodextrin (γ-CD) in a "host-guest" strategy, incubating it with PFAS molecules to form complexes. Due to its unique structure, γ-CD also functions as an "adapter" within the nanopore.
Through this design, interactions between PFAS and γ-CD in the nanopore system produce measurable current signal changes, facilitating the detection of perfluorooctanoic (PFOA) and perfluorooctanesulfonic (PFOS) acid molecules. By modifying the CD structure, specifically using 2-hydroxypropyl–CD (HP-γ-CD) as the host molecule, we achieved selective identification of PFOA and PFOS molecules, detecting PFOA, PFOS and mixed PFAS species in water directly at concentrations of 0.4-2 parts per million, which can be further reduced to 400 parts per trillion after pretreatment.
Additionally, the host-guest interaction between HP-γ-CD and PFAS molecules enabled us to identify broader PFAS families, including six perfluoroalkyl carboxylic acids (PFCAs: PFBA, PFPA, PFHxA, PFHpA, PFOA, and PFNA) and three perfluoroalkyl sulfonic acids (PFSAs: PFBS, PFHxS and PFOS). Alongside γ-CD and HP-γ-CD, we systematically investigated interactions between PFAS and α-CD and β-CD molecules within the nanopore system. Theoretical calculations and molecular simulations revealed the critical role of binding constants in these interactions.
Combining experimental analysis, ion transport modeling, and molecular dynamics simulations, this approach lays the groundwork for a portable device capable of real-time PFAS monitoring across diverse environments.
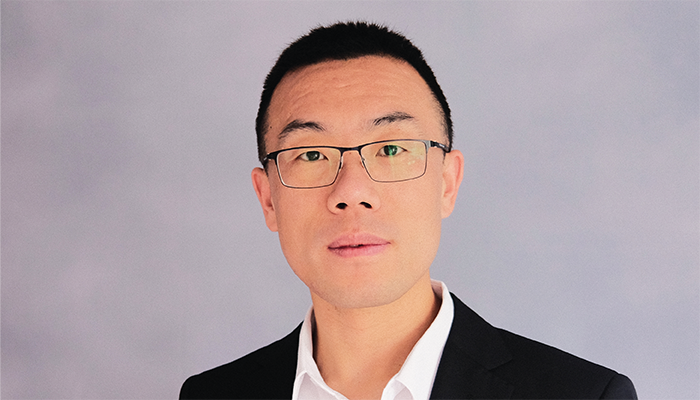
Xiaojun Wei
Credit: Supplied by Interviewee
What was the biggest analytical challenge you faced during your research?
We were initially excited to detect PFAS signals after incubating with γ-CD and PFOA or PFOS; however, we quickly realized that using γ-CD as an "aptamer" did not yield differentiated signals for PFOA and PFOS. Our challenge was to identify a suitable cyclodextrin to serve as an aptamer. After further investigation we found that by optimizing the cyclodextrin structure through chemical modifications, we could enhance detection sensitivity. Through continuous exploration, we selected HP-γ-CD as a new adapter, achieving differentiation and recognition of various PFAS molecules.
Was there a key breakthrough during development?
Our key breakthrough came from an unexpected observation rather than a deliberate attempt to create a CD-PFAS host-guest structure. In our lab, cyclodextrins (CDs) are commonly used as reference molecules to verify nanopore functionality. Initially, we aimed to detect PFAS molecules directly through the nanopore. However, we quickly discovered that adding PFAS molecules directly to the system caused the lipid membrane supporting the nanopore to break down, due to the disruptive effects of PFAS on the liposome. During one experiment, we noticed that the membrane's stability improved significantly when β-CD and PFAS molecules were both present in the system. This led us to consider that introducing a CD molecule could enable PFAS detection via the nanopore – a realization that was truly exciting! Our second “eureka moment” came when we replaced β-CD with γ-CD and observed distinct PFAS signals in the nanopore’s current readings, indicating successful detection.
How does your method compare with the "gold standards" of PFAS testing?
Our method offers a unique approach to PFAS detection by using a nanopore system with cyclodextrin as an adapter for PFAS recognition, providing a potentially rapid and cost-effective alternative to traditional techniques. Unlike the "gold standard" approach using liquid chromatography-mass spectrometry (LC-MS), which is highly accurate but also time-intensive and costly, our nanopore approach requires smaller sample volumes and aims to simplify the detection process as a whole. However, we currently face limitations in sensitivity compared to LC-MS, as our detection limit remains around 400 ppt, whereas LC-MS can reach a lower level to meet the latest EPA standards (4 ppt). Further optimization in our detection strategy is needed to achieve comparable sensitivity, but our method holds promise for field-based and rapid preliminary screening of PFAS in environmental samples.
What are the next steps for your research?
Our next steps involve enhancing detection sensitivity by optimizing testing conditions and designing new detection channels. This includes modifying the nanopore and improving the structure of cyclodextrins to achieve lower limits of detection, ideally meeting current EPA requirements. Additionally, we are working on differentiating and quantifying various PFAS types within mixtures, which will require the optimization of our experimental design as well as advancements in algorithms and data analysis.
The main barriers to more widespread adoption of this method will be the controllability and stability of the nanopore system. Currently, challenges include improving the long-term storage of biological nanopores and potentially replacing the lipid membranes that support these nanopores with synthetic materials to increase robustness.