Single-Molecule Mass Spec with Inertial Imaging
Conventional mass spectrometry can only measure the mass of a molecule – what if you could image the same molecule simultaneously?
John Sader, a professor at the University of Melbourne and one of the authors of a new study (1), says such MS imaging is now possible. Inertial imaging with nanomechanical systems (NEMS) was developed at the California Institute of Technology and could have a big impact on biomedical applications. Sader tells us more.
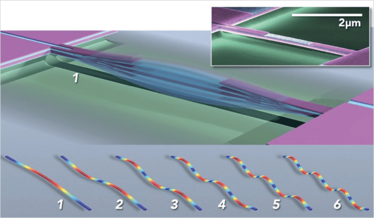
Multimode nanoelectromechanical systems (NEMS) based mass sensor; the main figure schematically depicts a doubly-clamped beam vibrating in fundamental mode (1). Conceptual “snapshots” of the first six vibrational modes are shown below (1-6), colors indicate high (red) to low (blue) strain. The inset shows a colorized electron micrograph of a piezoelectric NEMS resonator fabricated in Caltech’s Kavli Nanoscience Institute. (Credit: M. Matheny, L.G. Villanueva, P. Hung, J. Li and M. Roukes/Caltech)
How did you get involved in the project?
The work arose during a one-year sabbatical at Caltech. I’ve been collaborating with Michael Roukes’ group at Caltech since 2008, and spent the year (2012-2013, at Michael’s invitation) working closely with his team as the Kavli Nanoscience Institute Distinguished Visiting Professor of Physics. It was during this period that his group asked me if I could improve on the mathematical theory they were using to interpret their NEMS mass spectrometry measurements (a technique they pioneered). After some thought, I managed to dream up a new mathematical theory that was radically different and much simpler than the existing one. Rather than using a complicating nonlinear algorithm, my new theory only required adding up the raw frequency measurements, to measure mass – at first sight it seemed crazy that this should work, but it does (and there’s a theoretical proof!). It could also be trivially extended to a broader and new application: imaging the particle. This formed the basis for the inertial imaging work.
What is inertial imaging?
The idea is simple. Consider a vibrating taught string. When you pluck the string, and listen (and watch) closely, you’ll notice that it actually vibrates at many different frequencies (and vibration shapes). Each of these frequencies corresponds to a different string mode. It is the combination of these modes, and the frequencies they generate, which makes a guitar string sound different to a violin string. This is what musicians normally call ‘tone’.
Now, when a particle lands on the string, all of the string’s frequencies drop; the musical note sounds lower and the tone changes. These frequency drops depend on where the particle lands. They are largest if the particle lands on an anti-node of the vibrating string – zero on a node. But the nodes and anti-nodes of all the modes occur at different positions along the string. So when a particle lands on the string, the frequencies of its many modes drop differently – this gives a ‘frequency shift signature’ for the particle. It’s like a fingerprint!
The new theory interprets this signature by adding up the frequency shifts in a special way to measure the mass and shape of the particle.
What are the key benefits?
Conventional mass spectrometry only gives information about the mass of the particle or molecule. It cannot see what the particle looks like. In contrast, advanced microscopy allows the particle or molecule to be imaged, but not weighed. Our inertial imaging technology changes this paradigm by enabling an individual particle or molecule to be both weighed and imaged simultaneously. This gives a vital new piece of information that can be used for diagnostics.
For example in biomedical applications, mass spectrometry can be used to discriminate between different proteins, molecules or pathogens in general, based on their weight. But it is entirely possible that two different molecules will have very similar weights. Inertial imaging now enables the difference between these two molecules to be distinguished, by measuring their individual shapes. This can be done molecule-by-molecule, one at a time.
How does it compare with conventional techniques?
In conventional imaging, light (for example) is fired at a sample, and the reflection or scattering of that light is measured, which generates an image. While these techniques work fantastically well, there are some well-known drawbacks. One is that it is very difficult to directly image a particle that is much smaller than the wavelength of light. This is the so-called diffraction limit. It’s one of the reasons why electron microscopes are used, instead of light microscopes, to image very small nanodevices and nanoparticles – the size of these nanoscale objects is much smaller than the wavelength of light. Using electrons overcomes this barrier because their wavelength can be very small compared to that of light. But it can’t simultaneously measure mass.
While inertial imaging also uses waves – in the form of standing waves of an elastic beam – the wavelength of vibration plays no role in the imaging resolution. This is completely counterintuitive. The particle can be many orders of magnitude smaller than all wavelengths of vibration used – inertial imaging will work! Rather than the vibration wavelength limiting the measurement resolution, it is the inherent noise in the frequency measurement that gives the ultimate limit. But our analysis shows that this is no issue. The noise in current NEMS devices is sufficient to image particles with atomic-scale resolution. Specifically, our work shows that the minimal resolvable size using current carbon nanotube NEMS resonators is 0.3 nm – roughly the size of a silicon atom. We are working to experimentally demonstrate the ultimate limit of this new technology.
What are the next steps?
Our next step is to fully explore the ideas we reported in our paper. For example, we want to develop new NEMS devices specifically designed to optimize the performance of inertial imaging, explore the full potential of the new mathematical theory and algorithm, understand its intricacies, experimentally demonstrate the ultimate limits of its performance, and implement inertial imaging in real time mass spectrometry amongst many other goals. My collaborators at Caltech and Melbourne and I are all very excited about the possibilities.
- M. S. Hanay et al., “Inertial Imaging with Nanomechanical Systems,” Nat. Nanotechnol. 10, 339-344 (2015). DOI: 10.1038/nnano.2015.32
"Making great scientific magazines isn’t just about delivering knowledge and high quality content; it’s also about packaging these in the right words to ensure that someone is truly inspired by a topic. My passion is ensuring that our authors’ expertise is presented as a seamless and enjoyable reading experience, whether in print, in digital or on social media. I’ve spent seven years writing and editing features for scientific and manufacturing publications, and in making this content engaging and accessible without sacrificing its scientific integrity. There is nothing better than a magazine with great content that feels great to read."