In 1992, we launched Ocean Optics to sell what we described as the world’s first miniature spectrometer. It featured a very low-cost linear array detector that was originally manufactured for barcode scanners. We discovered this US$20 charge-coupled device (CCD) array detector significantly outperformed $1000 “scientific” photo-diode array detectors. The reason, we came to understand, is that the quality of microelectronics improves with quantity. High-volume manufacturing can use more expensive coaters and other capital equipment. Higher volumes allow for fine tuning and optimization of the process and implementation of more sophisticated quality control systems. The other breakthroughs we needed came from low-cost, high-performance optical fibers, and ever improving and lower-cost computers. These three components formed the basis for our fiber optic, PC-controlled spectrometer. The other breakthrough was a combination of marketing and engineering, two realms that have more common ground than you might think – both are experimental, involve solving problems, and treat the cost of a product as a design variable. We found that we could lower the cost of applying our spectrometer to new applications by making the system highly modular. Our goal was to make the creation of a new “product” or application possible without any additional engineering expense. Therefore, the components of a spectrophotometer (light source, sample compartment, spectrometer, readout and interpretation) were made and sold as individual items, coupled together with optical fibers to create systems.
The lower cost and modularity of our system opened up many new application areas, and also broadened our understanding of spectroscopy. We thought of our products as enabling optical sensing in general, not just classical absorbance and reflection spectroscopy – the same device could measure transmission/absorbance, reflection, elastic and inelastic scattering, interference, polarity, emission, and time-based changes. The affordability of the systems lowered the financial risk of trying out new types of measurements, and also helped in the adoption of new applications in the marketplace. Arguably, our marketing success broadened the awareness and appreciation of spectroscopic measurements and boosted the industry as a whole. One of our great successes was in education markets. I went through four years of undergraduate education with only a single day’s exposure to a Spec 20 in general chemistry lab. It even had a small sign pasted to it that said, “Do not touch the dials!” Today, many students at all levels (including high school) are using our spectrometers for experiments that include acquiring full spectra from their samples as .xls files, and learning how to manipulate and process data in a spreadsheet – valuable real-life skills. It is exciting to note that spectrometers identical to those that students are using have flown on space missions, been to the depths of the oceans and been used in field studies in all kinds of environments. In many institutions, the chemistry department shares spectrometers with the biology and physics departments, made possible by acquiring a few extra accessories.
We also helped develop many new optical sensing approaches. Our spectrometer enabled low-cost Raman spectroscopy, for example, which helped grow awareness of the technique and progress in methods and hardware to obtain cost-effective Raman data that was useful for specific applications. Our spectrometers were widely adapted for plasma chemistry applications, especially in electronics and optical vacuum process environments. We also had systems being used to look at absorbance of gases coming from volcanoes, smoke stacks, fence line monitors, automobile exhausts, marine sediments, exhaled breath and atmospheric pollutants. Our fiber optic reflection probe found great use in diffuse reflection of tissue for detecting cancers and other pathologies, and in backscattering measurements used to characterize particle size distribution in slurries, colloids and biological materials. Our spectrometer and a special connector brought spectroscopy to microscopes and telescopes. The spectrometers served as detectors for fiber optic chemical sensors including pH and O2, and even special sensors for monitoring the breakdown of solid rocket fuel in aging intercontinental ballistic missiles. The other important attributes of the systems we developed at Ocean Optics were small size and a low power requirement, which allowed portable spectroscopy for samples that can’t be brought back to the lab. We pioneered using laptop and notebook PCs and battery-operated light sources to make measurements in situ. More recently, our spectrometers have been added to autonomous vehicles and drones to look at spectral reflection of crops, natural vegetation, minerals and soils, algae in natural waters, and so on. The spectrometers add fine spectral information to coarse hyperspectral imagers and can be deployed for ground truth and sea truth measurements as well. Applications abound in agriculture for managing crops, finding invasive species in waterways, detecting fertilization, water stress, and ideal time to harvest.
The photonics industry is driven by advances in materials science and manufacturing technology. Detector development has been driven by the demand for higher-resolution and lower-cost cameras in cellphones and surveillance devices. The need for higher bandwidth communications has driven the development of better, faster lasers and detectors, higher-performance optical fibers, narrow wavelength filters for wavelength division multiplexing and a host of components for use in the near infrared (NIR) range. Better design tools, including optical modelling CAD software, have enabled improvements in all aspects of optical systems.
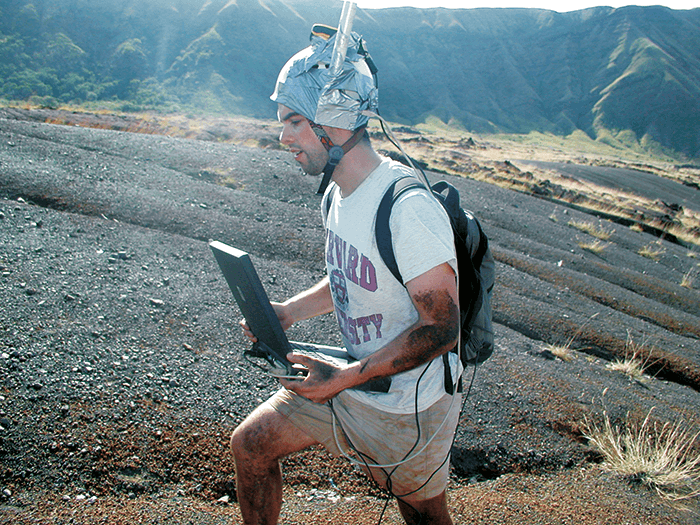
These photonics advances have benefited spectroscopy. Our miniature spectrometers are now available in the NIR-SWIR (short wave infrared) range; low-cost mid infrared (MIR) array sensors are now becoming available and will soon lead to the introduction of similar low-cost MIR spectrometers. The combination of pulsed high-speed lasers with very fast detectors in spectrometers has made another imaging technique possible – optical coherence tomography (OCT). In fact, the blending of spectroscopy and imaging is gaining traction with unique use of two dimensional camera sensors in a variety of hyperspectral and multispectral systems. New architectures of CCD arrays with two bins per pixel explicitly allow for measurement of fluorescence lifetimes in imaging systems. Advances in non-laser light sources have also been great for spectroscopy. Most significant is the expansion in LEDs, which are easy to switch on/off and can be operated at high speeds. LED sources are steadily reducing in cost, increasing in power and becoming available in an ever-broadening wavelength range (from deep UV to IR). As a result, the size and power requirements of spectroscopy-based systems have been reduced. In addition, narrow-band light allows for a narrow range of spectral measurements, with much less stray light. Our dream at Ocean Optics was to someday put a spectrometer in every home – to make spectroscopy a common and indispensable tool. The advance that may make that possible is the Internet, specifically the Internet of Things (IOT). The Internet provides a remarkable infrastructure for gathering, storing and providing data, analysis and interpretation to millions of users. To date, its capacity has been barely used. However, big data analytics, statistical analyses, AI and deep learning techniques are changing that. These new ways of getting answers are being used to overstep the traditional boundaries of instruments and measurements in rather unique ways. Today’s analytical models for tracking and predicting important phenomena may include data from many different instruments and sensors, as well as from the stock market, social media, remote sensing satellites and government agencies. In this new world, data is only judged by how it contributes to the solution. As such, optical data in unstructured situations may be just as valuable as more traditional setups. Recently, a research study was launched to use data from Apple watches to attempt to diagnose atrial fibrillation. That data being collected include heart rate and activity levels, but could also include other parameters, such as oxygen saturation, as well as data from social media, GPS location, air pollution, epidemiology of local rates of heart issues and so on. Now imagine if we swapped the two-wavelength sensor in the watch with a spectrometer; the possibilities are fantastic – not just a spectrometer in every home, but on every wrist! Mike Morris is President and CEO of Spectrecology and founder of Ocean Optics Inc, Florida, USA.
The most noticeable trend in analytical spectrometry is for compact, portable, embeddable, implantable, point-of-need instrumentation. Driven by smaller electronics, reduced power requirements and improved analytical performance, the most obvious example of this trend is the rapid evolution of compact Raman instruments. These handheld instruments are now found in law enforcement vehicles and airport security sites, where they are used for rapid field identification of a wide variety of chemical substances. Another example is how fluorescence imaging is taking on a whole new role in biochemical research. Solid state detectors and light sources (lasers and LEDs) play an important and growing role in all such instrumentation advances. Optical spectroscopic methods also lend themselves very well to remote sensing and there will always be new challenges in this realm. Satellite-based sensors now monitor a wide range of atmospheric and terrestrial species and provide essential data for tracking changes on our planet. One of my favorite examples is the NASA Aquarius satellite which, since 2011, has been mapping the salinity of the oceans using microwave emissivity, producing a map of the entire planet roughly once per week.
Changes are also happening in atomic spectrometry, where there are a number of specific challenges and opportunities. We only began making reliable quantitative atomic emission measurements about 100 years ago, but the past century has seen a continual, gradual progress towards lower detection limits, greater selectivity and wider dynamic range detection, with all sorts of new approaches. As with molecular methods, instrumentation is gradually becoming smaller and less expensive. Flame emission, spark and arc emission, flame and furnace atomic absorption and plasma emission have all played significant roles throughout much of the 20th century. All of these methodologies are now being replaced with ICP-MS.
In retrospect, it is rather obvious that mass spectrometric detection should eventually replace atomic emission, absorption and fluorescence, as ions are easier to detect than photons, and yield important isotopic information. ICP-MS approaches the ultimate limits of detection, is applicable to virtually every element, and exhibits very wide dynamic range. A huge range of analytical problems can still be served with the older techniques but it is clear that ICP-MS is already the method of choice whenever it is available. In addition, laser sampling has made possible increasingly refined, spatially resolved analysis on a wide variety of samples ranging from rocks to frozen tissue sections. In future, it is likely that more compact, efficient ionization sources will eventually replace the rather cumbersome ICP, and array detectors for ions will eventually make it possible to build compact mass spectrometers with a multiplex advantage. The purpose of any analysis is to solve a problem, and many problems lend themselves to spectroscopic solutions. For instance, generating new data for healthcare is undoubtedly one of the most important analytical challenges of the near future and spectrometric methods will surely play an important role. It is not hard to imagine a compact mass spectrometer with the power to provide a complete elemental and molecular profile from a small droplet of blood. Benjamin W. Smith is a Scientist at the Department of Chemistry, University of Florida, Florida, USA.
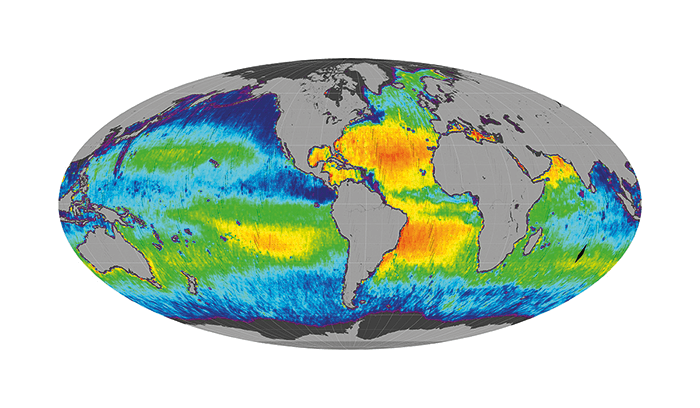
The field of analytical mass spectrometry plays an important role in so many areas. In the academic lab it is used to study and advance our understanding of basic questions in biological and physical sciences. Outside of the research laboratory it plays a key role in pharmaceutical development, forensic science, food analysis, and clinical diagnostics. It enables us to test our water to make sure it is clean, screen for explosives at the airport to keep us safe, and diagnose illnesses quickly and accurately. As scientists we should all serve a goal – to contribute to science and the betterment of our world. However, the specific aim of each spectroscopist is not the same, nor should it be. We need spectroscopists who are focused on the theory of spectroscopy and the continued advancement of our analytical tools. Fundamental research is critical to the forward momentum of science and technology. We have to understand our world before we can make it better. We also need spectroscopists who are focused on the application of these tools and the intersection of analytical science with other disciplines.
Instrumentation, such as quadrupoles, ion traps and time-of-flight are relatively mature – we are seeing incremental improvements each year but not the leaps and bounds of the past. In contrast, ion mobility spectrometry-mass spectrometry is an area in which we are just starting to realize the potential – there is great room for advancement and I can’t wait to see where this technology will go. Mass spectrometry is becoming available to the masses – the technology is becoming accessible to almost all scientific areas and is increasingly easy to use. I see this trend continuing with increased integration of mass spectrometry in many sectors. For example, the food industry is a space in which mass spectrometry could have a huge impact in the future. There is desperate need to improve the production efficiency, nutritional quality and safety of our food, and spectrometry can play a role in all of these areas.
The biggest practical hindrance right now is a lack of coordination around informatics – everyone is processing their data in different ways, which makes it very difficult to compare data across laboratories and/or instrument platforms. To solve problems like these, we need to train more analytical scientists, and those new scientists need to be from more diverse backgrounds – diversity drives innovation. Jessica Prenni is Associate Professor and Faculty Advisor of the Proteomics & Metabolomics Facility at Colorado State University, Colorado, USA.