Three Gurus of Spectroscopy
What has driven spectroscopic techniques into their current prominent position in a plethora of application areas? Here, Gary Hieftje, Peter Griffiths, and Volker Deckert combine over 120 years of experience to assess past successes – and failures – and to offer a glimpse of our light-filled future.
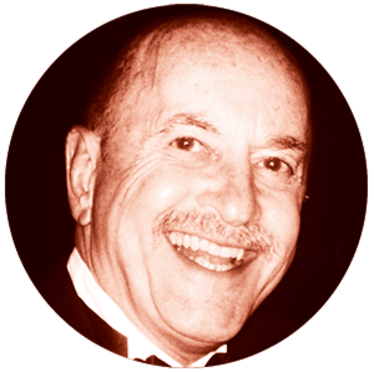
Gary Hieftje is distinguished professor and Robert & Marjorie Mann chair at the Department of Chemistry, School of Public and Environmental Affairs, and School of Informatics, Indiana University Bloomington, USA. Gary’s research interests include the investigation of basic mechanisms in atomic emission, absorption, fluorescence and mass spectrometric analysis, the development of instrumentation and techniques for atomic methods of analysis, on-line computer control, the use of time-resolved luminescence processes, and the use of stochastic processes to extract basic and kinetic chemical information.
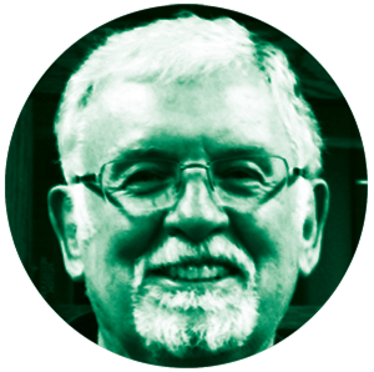
Peter Griffiths is emeritus professor of chemistry at the University of Idaho. His research interests involved many applications of vibrational spectroscopy in analytical chemistry. His group pioneered the use of diffuse reflection spectroscopy in the mid-infrared, the coupling of various types of chromatograph (GC, HPLC, SFC, TLC) to FT-IR spectrometers, surface-enhanced infrared absorption and Raman spectroscopy, and open-path atmospheric monitoring by FT-IR spectrometry. Peter was formerly editor-in-chief and is now second editor of Applied Spectroscopy.
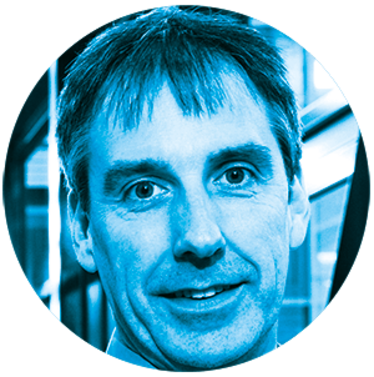
Volker Deckert holds a joint position at the Institute of Physical Chemistry at the University of Jena, Germany, and the Leibniz Institute of Photonic Technology, also in Jena. He obtained his Diploma and PhD from the University of Würzburg, Germany, working on difference-Raman spectroscopy. As a postdoc at the University of Tokyo and the Kanagawa Academy of Science in Kawasaki, he worked on non-linear and time-resolved laser spectroscopy of photo-induced isomerisation reactions. While at ETH Zurich, he started working on the development of high spatial resolution techniques for Raman spectroscopy, a topic which has followed him to Jena, where, in particular, he explores the possibilities of the technology to investigate structural changes of bio-related compounds with nanometer resolution
Where have the last 50 years taken spectroscopy?
GH: Generally, over the last 50 years we’ve been moving towards extremes: faster, smaller, higher resolution, higher power, lower noise. Indeed, improvements in instrumentation (including sources, detectors, and spectral-sorting devices) have been significant – laser technology is a major player there. Furthermore, increased coupling with other methods (separations, other spectrometric methods, electrochemistry, and so on), have pushed potential application boundaries.
More specifically, near-field methods have seen significant development. And, in terms of data collection, multiplexing (via multichannel systems) has given us the ability to capture entire spectra at once; multidimensionality has allowed us to take advantage of all the characteristics of electromagnetic radiation (frequency, amplitude, phase, polarization), and the dimensionality of information-rich methods, such as NMR, have been copied across various techniques.
VD: I think the main general trend at present is miniaturization, as noted by Gary. Instruments are getting smaller and smaller; hand-held spectrometers are a common sight at exhibitions. Another trend over the last 20-30 years has been the combination of spectroscopy and microscopy. This advance allowed for smaller sample volumes and different kinds of imaging techniques. Personally, I think this trend towards imaging really did the trick – seeing is believing! And distinguishing a compound from its direct neighbor directly without further invasive sampling is a major feature of today’s optical spectroscopies.
PG: Fifty years ago, the field of spectroscopy was dominated by atomic spectroscopy. I believe that it is fair to say that molecular spectroscopy has now assumed the more dominant role, largely because of the remarkable development of FT-IR, Raman and near-infrared spectrometers – and the many problems in analytical chemistry that require measurement of molecular (rather than atomic) spectra. Therefore, I will concentrate on molecular spectroscopies here. And in light of my training and expertise, I will largely narrow my responses.
What are the major impacts of spectroscopy – in analytical science or society?
PG: Developments in spectroscopy (both hardware and software) have allowed the composition of reactants, intermediates and products in industrial processes to be determined and controlled in situ, with high accuracy and in very short times. The result has been improved product reliability and decreasing costs. An obvious example of this can be seen in process analytical technology (PAT) in the pharmaceutical industry. We are also getting close to the point where several different forms of spectroscopy will be used for medical diagnosis.
VD: As a spectroscopist, I would like to say that spectroscopy plays a major role in both analytical science and society. After all, direct information about the molecular structure is possible. The truth is more complex though. Try to investigate a mixture and you learn to appreciate separation technology; and if you can afford to lose material, mass spectrometry can deal much better with mixtures as well. What is most interesting, in my opinion, is the fact that the different technologies in optical spectroscopy are constantly challenging each other in terms of sensitivity, spatial resolution and application fields. Whenever Raman spectroscopy came up with improved light sources or novel detector concepts, IR spectroscopy answered with Fourier transform technology or with focal plane arrays. Similar stories can be told for other optical spectroscopies and, as a result, we have now instruments that are tremendously more sensitive than 10-20 years ago – and, in many cases, specialists aren’t even needed to operate them.
GH: Let me take you back even farther than the first question. Seventy-five years ago, the only spectrometric method that was available in most routine-analysis laboratories was the Dubosc colorimeter (based on a technique invented by Jules Duboscq in 1870). Today, spectroscopic methods are pervasive and employ virtually all methods of spectral measurement (absorption, emission, fluorescence, scattering, acoustic, polarimetric, and others) and for a host of measurements (elemental, molecular, kinetic, chromatographic detection, remote sensing...). One of the most common methods of spectrometric measurement is the NIR method for measuring oxygen saturation via a probe clipped to a patient’s finger. Many other laboratory-based or bedside measurements also rely on spectrometry.
Many other sorts of common optical devices (polarized sunglasses, xenon and LED headlights, intruder-detection systems for home protection, collision-avoidance systems in automobiles, laser scanners at grocery checkouts, Lidar devices for measuring auto speed or passing through “red lights”, terahertz airport scanners) are all reliant on spectroscopy to some degree.
Becoming rather more abstract, several impressive natural phenomena – red sunsets, blue skies, the aurora, and lightning – are essentially dependent on spectroscopy!
Where are the strongholds in spectroscopy?
PG: Any list will omit important names, nevertheless, I’ll present some of the groups that are doing cutting-edge research in vibrational spectroscopy today. Raman spectroscopy: our fellow guru here, Volker Deckert at the University of Jena (TERS); Duncan Graham, University of Strathclyde (SERS, SERRS). Infrared Spectroscopy: Rohit Bhargava, University of Illinois (biospectroscopy, microspectroscopy); Alexandre Dazzi, University of Paris (nanospectroscopy); Naomi Halas, Rice University (surface-enhanced vibrational spectroscopy).
VD: There are definitely too many to mention here and you will probably get as many different answers as the number of people you ask... In terms of fluorescence spectroscopy, certainly the development of sub diffraction limit imaging in Göttingen or Janelia Farm are most impressive. Here in Germany, Stefan Hell (developer of STED) and Eric Betzig (developer of PALM) are the major protagonists. In these cases the technology could almost immediately be applied in biology and medicine. In other fields of spectroscopy, I would not speak of strongholds as such, the fields have matured over a long time, so there are many places doing high quality research.
GH: I can answer this best with a couple of (non-comprehensive) lists from the US. In the past: Illinois (Malmstadt), Michigan (Willard), Wisconsin (Walters), Iowa State (Fassel), Purdue (Pardue, Amy), North Carolina (Reilley, Eisenhour), Cornell (Morrison).
Today: Purdue (Lytle, Wirth, Simpson), North Carolina (Ramsey), Indiana (Hieftje), Illinois (Sweedler), Texas (Holcombe), Notre Dame (Dovichi), Arizona (Denton).
In terms of topics: near-field methods, imaging, atomic spectrometry, sensors, single-molecule sensing, nonlinear and two-dimensional methods, high-resolution techniques, excited-state kinetics, fast instrumentation, and correlation are all likely to continue to be hot.
How did those you have mentioned become so successful?
VD: In parallel with the increasing complexity of the molecules or systems that were investigated, the demand for higher time resolution or spatial resolution was a major driving force in instrumental development. In order to perform the desired, the researchers simply had to be several steps ahead of current commercial offerings. And with such advanced instrumentation, you can do things that others cannot do. Sounds easy, doesn’t it?! But perhaps it’s even easier than that – just like Terry Pratchett’s Discworld novels, where some people are hit by “Inspiration Particles”...
PG: I believe that in every case that I have listed above, the principal investigator has made significant advances in the theory of the technique in addition to the superb experimental achievements for which he or she is better known. It is not surprising that many of the top analytical chemists have training in physical chemistry, physics or an allied discipline. (Truth in advertizing: my doctorate is in physical chemistry!)
GH: Usually, I think success comes as a result of one or two highly innovative, driven faculty, whose new ideas and personalities draw colleagues and students to them.
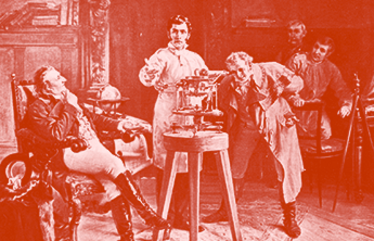
Joseph von Fraunhofer demonstrating the spectroscope. From a painting by Richard Wimmer.
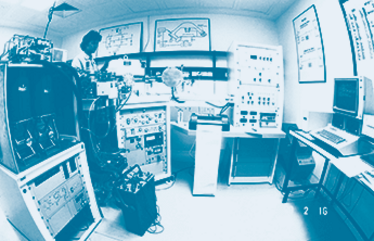
Mass-spectrometer (Finnigan MAT251) for measuring stable carbon (12C/13C) and oxygen (16O/18O) isotopes in carbonaceous shells.
Isotope laboratory of Alfred Wegener Institute for Polar and Marine Research, Bremerhaven, Germany. The engineer is checking the magnet for deflection and separation of the isotopes. The MAT251 was controlled by an Apple II computer (right).
Where are the great openings in spectroscopy?
PG: Perhaps the most important area in which vibrational spectroscopy is starting to show promise is medical diagnosis. Wong and his colleagues at the National Research Council of Canada showed that the mid-infrared spectra of exfoliated cervical cells showed evidence of extensive structural changes during carcinogenesis (1). Regrettably, the results were shown to be strongly dependent on the patient and it has taken many years of further work by a plethora of other research groups, of which I will single out the current groups of Max Diem at Northeastern University and Rohit Bhargava at the University of Illinois, to show that mid-IR and Raman spectra may allow various stages of cancer to be identified.
GH: The list of great openings is pretty long:
- Lasers
- Near-field methods
- Holographic gratings
- Charge-transfer array detectors
- Interferometers, FT methods
- Atomic absorption
- ICP emission and mass spectrometry
- Modern Raman spectrometers
- MCT detectors for IR
- Digital micromirror arrays
- Electro-optic and acousto-optic modulators.
VD: I would consider the wealth of applications that are now in reach all offer great openings. In particular, portable instruments that allow us to point spectrometers at virtually everything is probably the greatest opening.
How about dead ends?
GH: Dead ends? Interesting question… Widely tunable lasers seem to be one; though there have been many lasers, covering many wavelengths, none are easily tunable over a broad range. Diode-laser atomic absorption and fluorescence; great strides were made early, prompting some to predict that diode lasers would replace continuum sources, hollow-cathode lamps, and electrodeless discharge lamps for AAS and AFS.
However, lack of tenability and poor performance in the UV still make them largely impractical. Also, atomic fluorescence spectrometry, although fundamentally superior, has been killed by entrenched atomic absorption. And genome sequencing by individual base-pair optical scanning was simply too slow.
VD: Ask any scientist “how many dead ends?” in his or her career and the answer is likely to be, “more than great openings.” The problem with both great openings and dead ends is that you don’t really see them coming (ask Marvin Minsky about the confocal microscope...) Many ideas and concepts are worth trying, but in the end can prove to be flawed. The so-called aperture near-field optics concept to overcome the diffraction limit is such an example from my field. In principle, it can be applied to any optical spectroscopy, but sensitivity – and, more importantly, reproducibility issues – made it very difficult to handle. Interestingly, this dead end resulted in the development of a different concept: scattering or apertureless near-field optics, which can be dubbed as a great opening (so far!)
PG: One example of what appears to be a dead end is the non-invasive measurement of blood glucose by NIR spectroscopy. Well over $200 million has been spent on research to show the feasibility of such measurements without real success... Work is still continuing on this topic and some promising results are starting to be reported so, perhaps ultimately, this work may lead to a useful application.
I can also give an example of an incorrect result that has actually led to the development of an important technique – it can be found in the first report of surface-enhanced infrared absorption (SEIRA) (2). In this work, a thin layer of silver was deposited on the surface of a ZnSe internal reflection element and a few molecular layers of p-nitrobenzoic acid (PNBA) were deposited on the silver surface. After evaporation of the solvent, three strong bands were observed between 2800 and 3000 cm-1, which the authors ascribed to PNBA. Regrettably, the bands that were observed in the spectrum were due to aliphatic C-H stretching modes and PNBA has no aliphatic C-H groups. Nonetheless, this paper became “reference number one” in many subsequent papers on SEIRA…
What are the opportunities and challenges?
GH: There are plenty of challenges (or opportunities, depending on your point of view):
- Low-noise, wide-bandwidth single-photon detectors
- Tunable lasers for the entire UV-Vis wavelength range
- Broad-scene imaging at nanometer spatial resolution
- Bright non-laser sources for IR
- Low noise, ultra-wide bandwidth analog amplifiers
- The need to develop a method for measuring sample erosion on the nanometer scale.
PG: It may have become apparent from my response to the previous question, but I believe that both the biggest opportunity and challenge is in the field of medical diagnosis, especially cancer detection. The concept of being able to probe a tissue in situ rather than waiting several days for the result of a biopsy could revolutionize histopathology. From an instrumental standpoint, the developments in nanospectroscopy, i.e., the measurement of both mid-infrared and Raman spectra at a spatial resolution well below the diffraction limit, are very exciting. Deckert, our co-writer here, has reported the measurement of tip-enhanced Raman spectra at a resolution of better than 20 nm, so that the spectra of a few base pairs of a strand of DNA can be acquired, and several TERS instruments are now commercially available. In the last decade, AFM-IR measurements with close to this spatial resolution have been reported by Dazzi’s group in France (commercialized by Anasys Instruments in the USA) and by Keilmann and Hillenbrand in Germany (commercialized by Neaspec in Germany.) The challenge in TERS and AFM-IR measurements is to improve the spatial resolution to the atomic level; this will probably require smaller, more rugged AFM cantilevers as opposed to more sensitive spectrometers.
VD: Many optical spectroscopies are or can be made non invasive, which allows non-destructive detection or detection from a safe distance. These properties are ideally suited to medical diagnosis and many groups are currently developing systems for that purpose. Personally, I am most impressed by attempts in fast classification of bacteria by Raman spectroscopy. My colleague Jürgen Popp and his co-workers are developing strategies to identify bacteria on the strain level in less than two hours (something that takes several days of standard bacterial cultures). This advance really could change medical treatment of sepsis, when literally every minute counts. The biggest challenge here is likely to be the lack of synergy between scientific instrument development and the actual clinical environment. Physicians are used to robust and easy to use instruments. Spectral interpretation is definitely not on that list. Hence, apart from the actual instruments, automated interpretation and classification of the data is required.
How can spectroscopists continue to make a substantial contribution to science and/or society?
VD: The answer here is pretty much in the line with my previous statement and Peter’s earlier thoughts. I think medical diagnosis will have a big impact on the further development of spectroscopy and, hopefully, vice versa. But such developments need time and patience (good for patient safety, bad for rapid progress). Clearly, immediate breakthroughs in the field of clinical diagnosis are not to be expected. In view of changes in society, there is an increased need to remotely monitor patient status or perform quick screening in cases of emergency. Here, optical spectroscopy will surely have its fair share. After all, current smart phones already contain all the main parts required for spectroscopy: a light source and a detector. Include a laser pointer and you can do some basic fluorescence. The Star Trek Tricorder is almost here!
PG: Spectroscopists have never been very good at promoting their developments. Some important developments remain a well-kept secret while others are announced too early. Better ways of disseminating important developments in spectroscopy are clearly needed but I am not really the right person to say exactly how this should be accomplished. Perhaps that’s where The Analytical Scientist could help?
GH: We need to encourage new small instrument firms that employ breakthrough technology. In other words, the ongoing and increasing consolidation of instrument companies into large entities essentially discourages innovation. Let’s face it: there is little incentive for a large firm to adopt new technology that will compete with its own existing offerings.
And, by the way, the recent change in US patent law goes against this trend. Before, the demand was “first to invent”. Now, the US has followed most of the rest of the world in giving rights to the “first to file”. Unfortunately, this change legislates against innovation and publication by universities. The coin of the realm in universities is publications rather than patents; it compromises a student’s chances for a job and a faculty member’s chance for tenure if a publication is delayed until a patent is secured… But that’s another story
What dramatic improvements in spectroscopic instrumentation could you cite that have led to some of the current levels of performance?
PG: To answer this, I would like to go back several decades. The development of FT-IR spectrometers in the late 1960s and early 1970s allowed many of the limitations of grating spectrometers to be overcome. Today, nobody thinks about using a monochromator for mid-IR spectroscopy any longer, except as a polychromator in combination with a focal-plane array detector. Two developments in the 1980s can be cited as being largely responsible for the current popularity and high performance of Raman spectrometers: first, charge-coupled device array detectors replaced photomultiplier tubes and second, notch filters allowed the second monochromator to be eliminated. Today, hand-held FT-IR and Raman spectrometers are available with performance that would have been unthinkable on the bench-top only a few decades ago. I should also draw attention to the corresponding advancements in chemometrics and the development of sophisticated software that have enabled many applications that would otherwise be impossible on a routine basis.
VD: How far back do you want to go? Lasers are common today, but certainly without them most modern optical spectroscopies would be either impossible or extremely slow. Detector technology development has been somewhat more continuous; however, the introduction of CCD detectors to visible spectroscopy was a huge improvement. Quantum efficiencies of >90% and the ability to electronically record the whole spectrum dramatically increased the speed of detection. When used as pure imaging devices, the same advantages allowed for the fast acquisition of fluorescence images, for example.
Interestingly, the systematic combination of spectrometers and microscopes happened quite late in the case of infrared and Raman spectroscopy. In particular, the collection efficiency benefitted from the use of microscope objectives and, more importantly allowed mapping of larger areas and, consequently, the distinction of local variations.
GH: I would cite both array-detector technology and near-field methods as examples. Both now abound in commercial instrumentation and have dramatically improved performance and range of application. Similarly, nonlinear spectroscopic methods are being applied widely by biologists and biophysicists and are finding their way into the marketplace.
In the area of atomic spectrometry, key examples would be the improvement in plasma sources (for example, ICP, glow discharge, and more recently the atmospheric-pressure glow discharge), the development of electrothermal vaporization (L’Vov and others), the introduction of hollow-cathode lamps for atomic absorption (Walsh), and the invention of background correction methods for atomic absorption.
Do you see other landmarks on the horizon?
VD: If one could transfer the concept of energy dispersive detectors to the visible range with enough spectral resolution, it could be the most dramatic change in spectroscopy of all. You could get rid of the spectrometer itself as such a detector could directly detect the energy of incoming photons. I like that idea: spectroscopy without a spectrometer, as Rick Russo might say!
GH: Volker’s idea is a great one, and in fact was pursued by Tomas Hirschfeld during the time he was at Block Engineering. The problem, of course, is that in the spectral ranges used in “optical” spectroscopy, the photon energies are comparatively low, on the order of single eV. As a result, to obtain even modest resolving power requires resolution on the order of thermal energies. To my knowledge, Tomas didn’t employ cooled detectors, so the idea should be revisited.
Another invention on the horizon is the use of “fly’s eye” lenses for imaging and for miniaturization of spectrometric instrumentation. And, as mentioned earlier, the translation of the multi-parametric methods now common in NMR into the optical regime.
In the area of atomic spectrometry, people are still searching for a “better ICP”. It is becoming well recognized that the ICP is inefficient and error-prone, the latter caused by interelement (matrix) interferences. The causes for those interferences are finally being worked out, and methods for their detection, recognition, and control will follow. Similarly, the ICP requires lots of power, lots of argon, and generally substantial amounts of sample material. There are studies underway on small sources that operate in the open air or with air as the support gas, at modest power levels, that require only small amounts of sample, but which rival ICP performance...
PG: The development of quantum cascade lasers (QCLs) could open up new types of measurements in the mid-infrared region. At present, QCLs are expensive and the wavelength range is limited. But given a “killer app,” where hundreds or even thousands of instruments could be applied, the cost of these lasers could be reduced dramatically.
I have worked in the field of vibrational spectroscopy for 50 years and it has always amazed me that just about every year at least one major development has been reported. This trend will undoubtedly continue. I just wish that I was smart (and young!) enough to see what tomorrow’s developments will be.
- P. T. T. Wong et al., Proc. Natl. Acad. Sci., 88, 10988-10992 (1991).
- A. Hartstein, J. R. Kirtley and J. C. Tang, Phys. Rev. Lett., 45, 201-205 (1980).
Gary Hieftje is distinguished professor and Robert & Marjorie Mann chair at the Department of Chemistry, School of Public and Environmental Affairs, and School of Informatics, Indiana University Bloomington, USA. Gary’s research interests include the investigation of basic mechanisms in atomic emission, absorption, fluorescence and mass spectrometric analysis, the development of instrumentation and techniques for atomic methods of analysis, on-line computer control, the use of time-resolved luminescence processes, and the use of stochastic processes to extract basic and kinetic chemical information.
Peter Griffiths is emeritus professor of chemistry at the University of Idaho. His research interests involved many applications of vibrational spectroscopy in analytical chemistry. His group pioneered the use of diffuse reflection spectroscopy in the mid-infrared, the coupling of various types of chromatograph (GC, HPLC, SFC, TLC) to FT-IR spectrometers, surface- enhanced infrared absorption and Raman spectroscopy, and open-path atmospheric monitoring by FT-IR spectrometry. Peter was formerly editor-in-chief and is now second editor of Applied Spectroscopy.
Volker Deckert holds a joint position at the Institute of Physical Chemistry at the University of Jena, Germany, and the Leibniz Institute of Photonic Technology, also in Jena. He obtained his Diploma and PhD from the University of Würzburg, Germany, working on difference-Raman spectroscopy. As a postdoc at the University of Tokyo and the Kanagawa Academy of Science in Kawasaki, he worked on non-linear and time-resolved laser spectroscopy of photo-induced isomerisation reactions. While at ETH Zurich, he started working on the development of high spatial resolution techniques for Raman spectroscopy, a topic that has followed him to Jena, where, in particular, he explores the possibilities of the technology to investigate structural changes of bio-related compounds with nanometer resolution.