Nanotech Titan: Part Two
The toughest peer review? A group of investors around a table, says Chad Mirkin, who talks commercialization lessons learned and major discoveries: spherical nucleic acids and dip-pen nanolithography.
| 9 min read | Interview
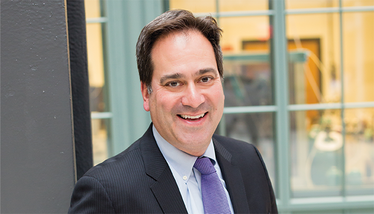
Credit: Chad Mirkin
Your discovery of spherical nucleic acids and the publication of your landmark paper must have been a big turning point in your career…
The paper you’re referring to – the one in Nature in 1996 – was a huge turning point. I believe it is the most cited Nature paper from the 1990s across all fields, which is amazing, right? Even 30 years later, it still garners a couple hundred citations a year and has over 8,500 citations. The reason it was so foundational is that it introduced this entirely new concept of “programmable atoms,” which redefined how we think about chemistry. We showed that you could take particles and, by attaching DNA to their surfaces, transform them into building blocks with bonding properties determined by that DNA.
It also became clear that this arrangement of nucleic acids on a particle created a new form of DNA and RNA – a structure with no natural equivalent and with unique properties. We initially pursued this as a basic scientific question, but it quickly became apparent that this new structure could have practical uses. We weren’t developing it with diagnostics or therapeutics in mind, but as we studied how it behaved, new possibilities emerged: the particles would aggregate when binding to complementary DNA, creating distinct colorimetric changes. Suddenly, we could develop colorimetric DNA and RNA sensors. Then, we discovered they could enter cells without needing transfection vehicles, which opened doors to cellular diagnostics and even manipulating cell behavior for therapeutic purposes.
This whole journey was about exploring a new form of matter and understanding its unique properties. I often say we “repurposed the blueprint of life,” and that allowed us to solve some big technological challenges.
And what about the dip-pen? How did that discovery come about?
The story behind dip-pen nanolithography (DPN) is interesting because it was somewhat accidental. We were initially studying the topology of polymer surfaces. My postdoc, Richard Piner, and I had very different approaches to science – I’m the intense “go, go, go” type, while he was more laid back. One day, he left the atomic force microscope (AFM) in contact with a substrate and went out for a smoke break. When he came back about 30 minutes later, he did a survey scan in lateral force mode and noticed what looked like a small droplet forming under the tip. It eventually faded into the background, but what he’d observed was the meniscus effect for the first time.
After further study, we found this effect to be dynamic. Water would move either up or down the tip, creating either recessed layers or additive layers on the surface. Richard was thrilled – he’s a physicist, and physicists love observing these kinds of phenomena. But as a chemist, I wasn’t as impressed by water transport. I told him, “If you want to impress chemists, make something with it.” So, we decided to put molecules on the AFM tip and use a surface, like gold, where these molecules could chemically anchor. This way, we could get stable layers rather than metastable ones. That was the birth of dip pen nanolithography.
We started with alkane thiols on gold, but any interaction pair could work. It turned out to be the first form of nanoscale additive manufacturing because, instead of using AFM as a reader, we were now using it to synthesize and structure surfaces. DPN has since evolved – today, it doesn’t use a single AFM tip but millions of elastomeric tips on a glass substrate, each capable of independently synthesizing something. It’s now the most scalable form of synthesis on the planet.
On a two-by-two centimeter chip, we can synthesize up to 56 million new materials. To put that in perspective, all scientists combined had made and characterized about a million new inorganic materials before this. Now, in one experiment, we can make orders of magnitude more materials. That was an “aha” moment – a realization that this could change the world. I think the resulting paper has more than 4,000 citations and National Geographic even named it one of the top 100 discoveries of the last century.
What makes DPN so powerful is that it’s not just about making new materials quickly; it’s about exploring combinations that were previously unimaginable. For example, we can create materials as catalysts for water-splitting reactions, helping replace scarce and geopolitically sensitive elements like iridium. Using this tool, we can explore millions of materials to find those that are as effective as iridium in catalytic reactions but without its limitations.
The difference, I think, between people who succeed in science at a high level and those who don’t often comes down to recognizing those moments and knowing what to pursue. The ability to recognize that something is worth spending time on is a big part of the talent required to push science forward.
What excites you most in your current scientific research?
First and foremost, I'm really excited about structural nanomedicine. I think it's the future, and I believe it has the potential to be transformative across the scientific community. The challenge is creating complex medicines that are structurally well-defined, where we can use analytical techniques to verify their design and employ AI to help optimize the composition, reducing the massive combination space needed to get to medicines that are not only chemically precise but optimized for efficacy and minimal toxicity. These materials would allow us to target diseases that current small molecules and biologics just can’t tackle. Structural nanomedicine is really an exciting frontier.
The second area that’s really crucial is using the high-throughput synthesis tools we’ve developed to create an inflection point in materials discovery. This could revolutionize the way we, as scientists and engineers, find materials that make a difference. We’re talking about new catalysts, superconductors, battery materials, display technologies – you name it. Our ages of progress have always been defined by the materials we could access: the Stone Age, Bronze Age, Iron Age, Silicon Age, even today’s advanced alloy age. When we discover materials that redefine these standards, we unlock new possibilities for innovation. That’s why I’m so enthusiastic about the high-throughput synthesis tools powered by techniques like parallel polymer pen lithography and beam pen lithography. I think they’ll be the driving force for materials discovery and scientific advancement for decades, if not centuries, ahead.
We’ve spoken about science and engineering. But what about commercialization?
Well, that’s a challenging shift, and it really selects for a particular skill set – someone who can handle a lot of "no’s." It turns out that just because you have a good idea, people won’t immediately open their checkbooks. And a lot of what determines success is outside a scientist’s control, which is difficult. Scientific discovery is the foundation, but after that, you need the right team on both the engineering and business development sides – people who can execute commercially. And access to those people is crucial. Then you need investors who believe in you and who are willing to take a chance. This is a whole different ecosystem from the scientific side.
Early on, it’s especially tough. I remember going over to Kellogg, our School of Business, knocking on doors and saying, “I have this idea for a new, powerful form of medical diagnostics based on DNA and RNA detection.” I needed two things: business students who could research the market landscape to see where this fit and a business plan to understand what it would take to move from idea to reality. Scientists don’t always realize people won’t just invest in a good idea. Investors want to know the need it addresses, the size of the market, whether it justifies the investment, and how long it will take.
So, you’re using a completely different skill set and need to be your own biggest critic. Even if you’re convinced of your idea, you have to convince everyone else, and that usually means a lot of naysayers. But you only need one person to say "yes" to get started. After that, the clock starts ticking: you use the funding to prove your technology, de-risk it, and show you’re building something commercially viable and worth further investment. It’s a relentless process, but if you get it right, it’s extremely rewarding.
Do you find that going through that process hones your scientific rigor as well?
Absolutely. Building a business and doing science the right way share a lot in common in terms of approach. I think it makes you a better scientist when you critically examine the potential impact of your technology and refine your model aggressively. It’s about not buying into your own hype; I know how to make things sound great, but I prefer to focus on things I genuinely believe are great because I’ve vetted them rigorously. Then I feel I can defend them at the highest level. Sometimes, of course, you don’t have all the answers, but being aware of the possible weaknesses helps you think about the questions or experiments needed to address them.
When we start a new company, we’ll spend a year or more just vetting the concept, asking, “Why won’t this work?” and gathering a team to challenge it. We make sure we have data to counter each criticism we might face. That process is essential, whether it’s a scientific hypothesis or a business venture, and it sharpens your focus in both areas.
How do you balance self-confidence and self-criticism?
You have to be confident, right? I’m a very confident guy, but confidence without being self-critical is just hallucination. So when you’re doing science well – or building a high-tech business – you have to challenge your ideas and have solid answers for all the potential critiques because you will definitely hear them. And the toughest form of peer review isn’t a journal article; it’s a group of investors around a table. In that case, it’s not just about doing a good job; if an investor backs something that fails, they could lose everything.
Chad Mirkin is Director of International Institute for Nanotechnology & George B. Rathmann Professor of Chemistry, Professor of Chemical and Biological Engineering, Professor of Materials Science and Engineering, Professor of Biomedical Engineering, Professor of Medicine, Northwestern University, USA