The problem
Standard gel electrophoresis has been intensively used for DNA fractionation in various genotyping and sequencing applications, and has the advantages of great simplicity, versatility, and reproducibility. However, it suffers from long processing times – many hours or even days; for instance, a typical pulsed-field gel electrophoresis (PFGE) device takes 25 hours to perform fractionation of 5–120 kbp DNA. Given trends towards second-generation sequencing, replacing standard gel electrophoresis with microchip-based systems is an attractive option to minimize processing time and optimize DNA fractionation. We set out to develop a new method to improve sample throughput and recovery compared with current technology.Background
The development of DNA electrophoresis, using both slab gels and capillaries, enabled the first-generation DNA sequencing and genotyping technologies required for the Human Genome Project. However, only minimal sample numbers could be separated and identified, at very high cost. In recent years, efforts have been directed towards reducing the cost and analysis time of DNA genotyping in second- and later-generation sequencing tools. To achieve this, sample preparation methods, such as electrophoresis, must be optimized to increase the throughput and efficiency of the analyses via user-friendly, portable and functional platforms. In some devices, traditional DNA separation gels have been replaced by microfabricated post arrays for separation of genomic-length DNA. However, defect-free fabrication of 3D nanostructures, such as nanopost arrays and crystalline nanoarrays, is extremely challenging. 2D nanostructures are easier to manufacture, but intrinsically yield low sample throughput. An ideal sieving matrix should have simple design and fabrication steps, yet provide high-resolution, high-throughput separation. And that’s why we opted for gel-based devices. People had originally considered continuous flow separation in such devices impossible, but we showed that it could work.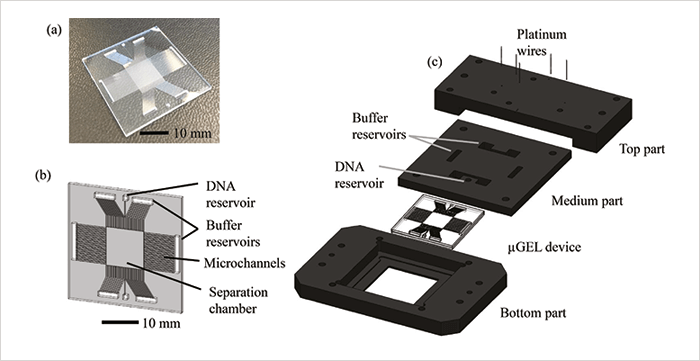
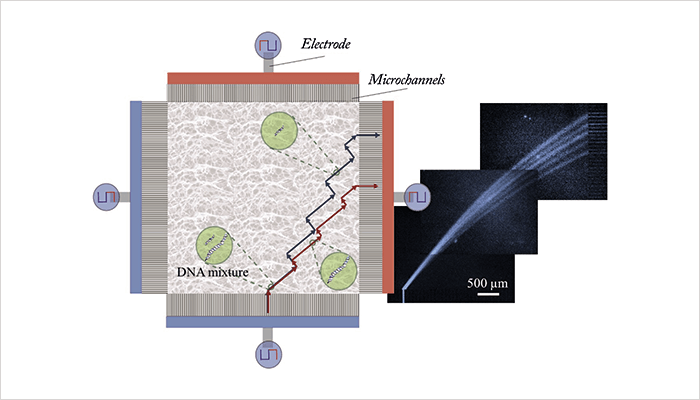
The solution
In the classic DNA electrophoresis approach, an electric field is continuously applied in one direction in a gel matrix and DNA fragments with different base pair numbers (different sizes) are separated in that direction. In PFGE, electric fields of the same strength are switched between two different directions – larger DNA fragments are slower to realign when the field switches, so the fragments become separated by size, but it can be a slow process. In our microchip, we use alternatingly applied orthogonal electric fields of different strengths. Larger fragments will move more slowly than smaller ones in the low field, but both sizes will move at the same speed in the high field. As a result, separation is achieved in the low-field direction and the DNA molecules are rapidly transported through the chip by the high field. The DNA molecules don’t all move in the same direction, as in the classical 1D separation, but in various size-dependent directions somewhere in between the two applied field directions (a snake-like motion). Our new chip separates DNA fragments within a few minutes, in high resolution, and purifies the fragments by separating contaminant salts from the fragments. The chip is made of glass and has a square separation chamber of 1 cm2, with microchannels on the sides to properly direct the electric fields. The chamber is filled with agarose gel, a standard medium for DNA separation. There are also reservoirs for sample injection and electrodes for applying the fields (see Figure 1). The chip is easy and cheap to produce using basic cleanroom techniques. It is also versatile, as the type and concentration of gel and the properties of the electric fields can be adjusted to the application.From the beginning of this research, we focused on using a “continuous flow separation” method for fractionating DNA. However, getting to the final concept was a process of trial and error. First, we tried to separate a DNA ladder in a polydimethylsiloxane (PDMS) device with T-shaped separation channels, by applying increasing electrical field in one direction. The device was filled with different agarose gel concentrations to achieve a direction-dependent velocity; however, we could only achieve baseline separation for two different fragment sizes, so it was back to the drawing board. Next, we tried to apply an electric field continuously in an agarose gel-filled 2D separation device in two directions without changing the field strength. Lower electric field strengths did not give successful separation owing to diffusion and frontal separation effects. Neither did applying higher electric fields, due to the similar mobilities of small and large DNA fragments. But by combining the two, we could separate DNA without band broadening problems at low electric field strengths, and simultaneously drive the DNA fragments towards the outlet by applying higher electric field strengths, resulting in a method that unites speed and good resolution. The potential was obvious.
However, before realizing our idea we had to address a few challenges – including designing a 2D separation matrix that could enable the generation of parallel electric field lines during the separation. In separation matrices with uneven electric field distribution, the separation cannot be performed because DNA fragments will always follow the field lines. This was solved by the addition of the side microchannels. Another great challenge was to produce a single device to cover the separation of a wide range of base pair sizes. In our device, pore size of the gel determines the base pair range in which good separation resolution is achieved, unlike previous DNA separation platforms where isotropic arrays were created using micron-size post arrays (for very long DNA separation), or colloidal crystals (for shorter DNA separation). However, redesigning the post arrays to the base pair range of interest is both laborious and expensive. We solved this problem by basing our designs on the use of inexpensive hydrogels, the pore size of which can be easily adjusted by changing the concentration.
Beyond the solution
The idea of introducing a new separation mechanism to the mature field of gel electrophoresis is exciting. I believe the technology has great potential to be commercialized; for example, it could help diagnose genetic disorders using a much smaller sample volume compared to conventional devices. We believe the microchip will be of broad interest for next-generation sequencing and clinical diagnosis applications, as it can achieve similar separation resolution in much shorter separation times compared to currently available devices. In particular, detection of pathogenic diseases by sorting pathogenic nucleic acids would open new avenues for the market. The device is designed to serve for sample preparation, which is crucial to eliminate false positive/negative results. Importantly, applications of the microchip can further be extended to protein gel electrophoresis by replacing the agarose gel with polyacrylamide gel.References
- B Gumuscu et al., “Exploiting biased reptation for continuous flow preparative DNA fractionation in a versatile microfluidic platform”, Microsystems & Nanoengineering, 3, 17001 (2017).