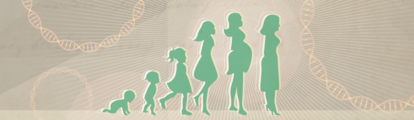
Mass Spec at the Analytical–Clinical Interface
The fight against disease is in no way an easy one – could the power of mass spectra prove to be mankind’s secret weapon?
Matt Hallam, Candice Z. Ulmer, John Yates, Donald Chace, Peter Nemes | | Longer Read
The role of MS in screening, monitoring, and subsequently improving patient health has far-reaching implications – from giving premature babies a fighting chance to evaluating environmental factors that contribute to disease. These applications span the clinical research spectrum, from the operating room to the lab bench, and – thanks to instrumental advances and the increasing power of data processing methods – are becoming increasingly important. Here, four influential analytical scientists – Candice Ulmer, John Yates, Donald Chace, and Peter Nemes – detail how they are wielding the power of mass spectra to identify, support and treat patients now and in the future.
Omics and Public Health
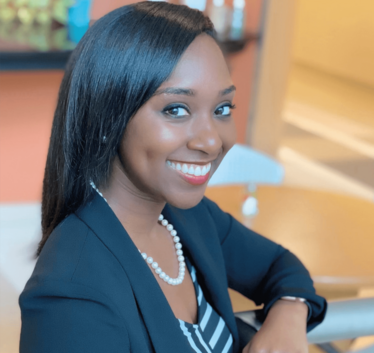
By Candice Z. Ulmer, Research Chemist & Associate Service Fellow, Clinical Chemistry Branch, Centers for Disease Control and Prevention, Atlanta, GA, USA
As a Clinical Research Chemist at the Centers for Disease Control and Prevention, my responsibilities include the planning and execution of programs for the harmonization and accurate reporting of chronic disease biomarkers – and other biomarkers deemed important by stakeholder organizations, including parathyroid hormone (PTH), anti-müllerian hormone, follicle stimulating hormone, luteinizing hormone, and steroid hormones.
We study these markers to enhance the diagnosis and treatment of select endocrine diseases and advance CDC Standardization Programs – aimed at improving the accuracy and precision of these same measurements, and many more, in patient care. In fact, I now ensure the accurate reporting of 17 such analytes, including the examples mentioned above, in addition to further roles generating population data, training laboratory professionals, and acting on the International Federation of Clinical Chemistry and Laboratory Medicine Committee on Bone Metabolism and the Clinical Chemistry Committee for the American Society for Mass Spectrometry.
A current focus of my research is the development of a reference measurement procedure for the accurate quantitation of PTH and its related fragments by ultra-high-performance LC-high-resolution MS. This method currently serves as the only top-down proteomics method for PTH quantitation, with the lowest limits of quantitation for an MS-based method (10 pg/mL). We apply this approach to measuring PTH, which is a key biomarker in the diagnosis and treatment of chronic kidney disease-mineral and bone Disorder, hypo- and hyperparathyroidism, hypercalcemia, and vitamin D deficiency. Currently used tests suffer from issues with sensitivity, PTH instability and measurement variability, while MS allows for the accurate measurement of this analyte over four orders of magnitude in serum and plasma with a high specificity. PTH also has many fragments with potential clinical relevance, which may go unconsidered without MS-based measurement.
I’ve also conducted significant work in MS-based lipidomics. In this work, I developed multi-omic metabolomic and lipidomic methods, including their translation into biomedical and environmental applications to predict risks to human health. Notable projects include monitoring the effects of environmental exposures on human and marine life, predicting health risks for diseases, such as type 1 diabetes and melanoma, as well as the first interlaboratory lipidomics study, which assessed lipid measurement variance to develop a standard reference material.
As for the future, I expect to see MS incorporated into a number of broader clinical applications. A key element of this will be the transition from single analyte biomarker assays to quantitative panels able to measure multiple biomarkers and thus assess numerous disease states simultaneously.
The Therapeutic Promise of Proteomics
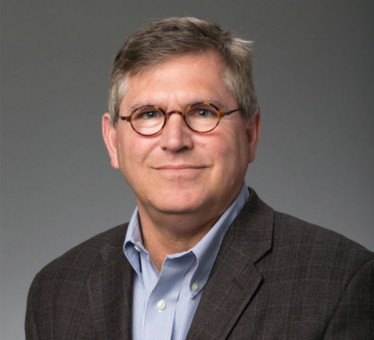
By John Yates, Professor, Department of Molecular Medicine, The Scripps Research Institute, CA, USA
The use of MS in clinical chemistry is growing rapidly. It is already the dominant clinical method in a limited number of applications, including newborn screening for metabolic disorders and bacteria identification, and many other applications, such as vitamin D and insulin-like growth factor-1 testing. These tests are being increasingly being performed with MS. A broader implementation of MS technology in a clinical setting may require a “killer” application that cannot be achieved with other methods – 3D proteomics may be just that application.
Most disease diagnostics are currently based on the presence/absence or increase/decrease of target molecule(s) – often a protein. Yet, the development of 3D diagnostics, where diagnosis is based on disease-induced conformational changes, may improve test specificity. 3D proteomics, an MS-based method that measures protein conformations on the proteome scale, seeks to determine whether disease status or associations can be predicted based on these conformations, and whether they can be reliably measured.
Protein misfolding drives many diseases, including Alzheimer’s, Lewy body dementia, cystic fibrosis, and cancer, but our current understanding of this comes primarily from in vitro measurements of protein structure. We are pursuing an improved understanding of in vivo protein misfolding in these diseases with MS-based 3D proteomic methods, which quantify protein conformations using surface accessibility and cross-linking. My laboratory is currently studying in vivo folding of the cystic fibrosis ion transport regulator (CFTR) when affected by various non-trafficking mutations, and under different kinds of rescue conditions, including drug treatment. A number of analytical techniques have contributed to this research, but the implementation of 3D proteomics to examine CFTR conformation in the context of the whole in vivoproteome promises to significantly advance our mission, opening doors to conformational 3D diagnostics.
Despite the utility of a 3D proteomics strategy, its scale remains limited today. Improvements in MS and related software for mapping quantitative MS data onto crystal structures have been helping to drive the technique’s applications, but getting 3D proteomics to work across entire, complex proteomes is a work in progress. In addition to the diagnostic potential of these methods, they provide important insights into the basic biology associated with protein misfolding diseases – and new avenues for structural drug development.
Other conformational methods are being developed; certainly, we are not the only ones working on this approach. This is a growing area with great potential. To date, conformational diagnostics have been implemented principally through antibodies designed to bind to specific protein conformations or contrast agents used in imaging methods. Conformational antibodies are tricky to make and imaging methods provide confirmation of disease. Diagnostic methods based on protein conformations using methods like 3D proteomics in an easily obtained biofluid like plasma could potentially provide earlier diagnosis, if protein signatures can be found. Within the next ten years, we hope to provide a new parameter for the proteome by measuring its conformational alterations as a function of disease.
Every Second Counts – Newborn Mass Spec Screening

By Donald Chace, Chief Scientific Officer at Medolac Laboratories – one of the primary developers of newborn metabolic screening using tandem MS
My main focus has historically been metabolite identification – detecting rare, inherited metabolic disorders through “newborn screening.” In fact, my team developed a tandem MS technique for the detection of phenylketonuria – an inherited metabolic disorder caused by the toxic buildup of phenylalanine in the blood that can lead to nervous system damage – from newborn dried blood samples. Our method works by analyzing the phenylalanine:tyrosine ratio within 24 hours of birth. We have since expanded these tests to study further inborn errors of metabolism.
Confounders complicate the task, however; many false results in newborn screening are due to abnormal metabolite elevation not caused by inherited disease – especially in premature, very-low-birth-weight infants. Why? In part because of immature systems, and in part due to the practice of nutrition, where the goal is to get an extraordinarily tiny baby to grow – either of which can result in abnormal metabolism and toxicity that mimics metabolic disease. The goals of our research have been to define baseline values and understand what causes abnormal profiles that are not of genetic origin.
Because our MS method is used to measure multiple metabolites in newborn screening, it is the first true metabolomic application. And the data processing is as essential as the method itself. Each metabolite detected requires quantification and standardization (using stable isotopes), which converts an ion signal intensity to a relative ratio, and finally to a concentration. Once completed, it is referenced to population means and cutoff ranges. Because each disease exhibits a different pattern, these concentrations must be interpreted from metabolite concentrations relative to one another (a key example being the phenylalanine:tyrosine ratio mentioned earlier).
High-performance LC can also be used for such approaches, but the tandem MS screening approach I developed ditches the chromatography, which takes longer per analysis and makes high-throughput analysis challenging.
Dried blood samples offer a number of advantages, including simplified transport, lower risk of infection, and the low volumes needed (around 10 microliters, compared with around ten times that volume from a heel stick). Such a difference is, of course, highly significant in the case of newborns.
Looking forward, I’d suggest that correlation of blood chemistry acquired by MS with genetics and lifestyle choices will allow us to identify optimum nutrition for growth and help avoid fat storage, marking the beginning of a true age of personalized nutrition – and medicine.
Understanding Development, One Cell at a Time
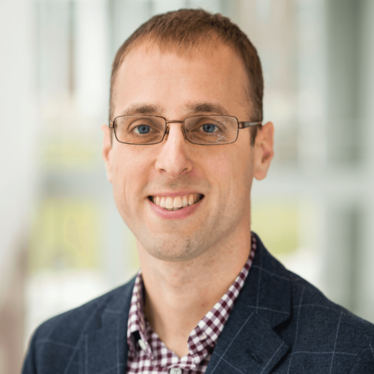
By Peter Nemes, Department of Chemistry & Biochemistry, University of Maryland, College Park, MD, USA
My lab builds microanalytical MS instruments to study how cells orchestrate molecular programs as they give rise to complex tissues, organs, and organisms during normal and impaired development. Understanding these mechanisms is key to identifying biomarkers and developing specific and sensitive assays and therapeutics – all key to advancing personalized medicine. The molecular mechanisms uncovered could, for example, be used to grow tissues or organs for clinical applications on demand.
We have constructed ultrasensitive instruments to measure metabolic and proteomic reorganization in single differentiating cells and cell clones in the South African clawed frog (Xenopus laevis) and zebrafish, as well as single neurons and functionally important nuclei in the mouse brain. By conducting such experiments, we are unraveling the incredible complexity of development at the level of single metabolites’ effects on embryonic cell fate and organogenesis. In fact, our MS approach allows the acquisition of single-cell omics data across the entire embryo; we were recently able to identify chemical differences underscoring embryonic patterning during Xenopus development.
How do we do it? We perform microdissection or use fine-fabricated capillaries to collect very small quantities of material from individually targeted single cells in live embryos. These custom-built, electrophoresis-based instruments are able to analyze samples as small as 1/10,000th the size typically required for contemporary spectrometers and related analytical sample processing workflows; our new-generation electrospray ionization interfaces effectively ionize these biomolecules with ~200 zeptomole sensitivity. Molecular compositions identified by MS then allow us to form hypotheses, which we test through functional biology experiments.
The details unveiled will hopefully open the door to inducing specific types of tissues for medical applications in the future. To help maximize the impact of our studies on translational research, we collaborate with numerous experts in cell biology, developmental biology, cancer biology, genetics, animal sciences, neuroscience, and so on – in both industry and academia. We have several projects on understanding the molecular aspects of cancer and impairment in embryonic patterning, hearing, vision, circadian cycle, and stress. Alternatively, the information we extract may allow us uncover molecules in the body (for example, natural metabolites) and in the environment (for example, toxins) that may impact embryonic development.
Our research brings many challenges – from maintaining a healthy colony of live frogs (!) to building and using complex MS instruments. But the potential knowledge to be gained – and its vast applications in medicine and elsewhere – mean that we relish facing those challenges head on.
Besides conducting our own original research using our technology, we also want to empower other investigators who can benefit from ultra-sensitive measurements. And so, it is highly rewarding to see that our tools and approaches have been adopted or adapted by several laboratories thus far.
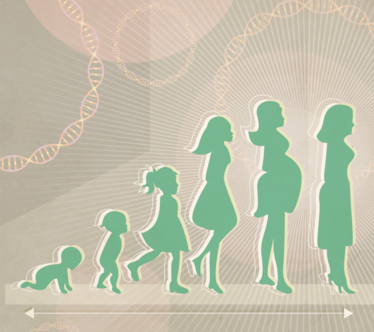
I've always wanted a job that fosters creativity - even when I worked on the assembly line in a fish factory. Outside work, I satisfy this need by writing questionable fiction. The venture into science writing was an unexpected departure from this fiction, but I'm truly grateful for the opportunity to combine my creative side with my scientific mind as Editor of The Analytical Scientist.
Research Chemist & Associate Service Fellow, Clinical Chemistry Branch, Centers for Disease Control and Prevention, Atlanta, GA, USA
John Yates is Ernest W. Hahn Professor of Chemical Physiology and Molecular and Cellular Neurobiology at Scripps Research, LaJolla, California, USA. He was recently named Editor of the Journal of Proteome Research.
Chief Scientific Officer, Medolac Laboratories, Boulder City, Nevada, USA
Department of Chemistry & Biochemistry, University of Maryland, College Park, MD, USA