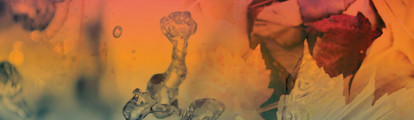
Testing the Water
DBPs – disinfection by-products – could pose an underestimated threat to our health, wildlife, and environment.
Susan Richardson | | Longer Read
When leaves fall into rivers and lakes, they get broken down by insects and microscopic organisms into matter that dissolves in the water. The resulting organic cocktail is mostly humic acid (up to 70 percent) - plus fulvic acid and pieces of dead bacteria and proteins from different living organisms. The water from the rivers and lakes enters your local drinking water plant and is disinfected to kill any harmful pathogens and make it microbially safe to drink. However, the organic matter in the water reacts with these disinfectants to form disinfection by-products (DBPs).
DBPs are diverse in nature. For example, coastline cities may have intrusion of sea water into freshwater supplies, which introduces sodium chloride, sodium bromide and sodium iodide into DBP formation (brominated and iodinated compounds are typically more toxic than those with only chlorine). DBPs are also effected by anthropogenic (man-made) contaminants, which are typically introduced from (inadequately) treated wastewater.
Most cities are downstream of another city’s wastewater, and not everything is removed in wastewater treatments; in fact, they are not designed for that purpose. For example, we have discovered very toxic iodinated DBPs produced from medical imaging compounds; iodoacetic acid is the most genotoxic DBP ever found – twice as toxic as bromoacetic acid. Indeed, X-ray contrast chemicals are the least removed pharmaceuticals in wastewater.
In the USA, we remove pretty much zero percent; in Europe, it’s maybe 20 percent. These compounds are specifically designed to be very stable in the body, so that they’re non-toxic to people. But they are injected in huge amounts – 200 grams intravenously for soft-tissue imaging. What happens to them? They are excreted by the patient, go down the toilet, through the wastewater treatment plant and out to the river – completely untouched. If you live in the city downstream and your plant chlorinates the water (which most of them do), you may well encounter these highly toxic DBPs…
Given the scale of the problem, I’m surprised that DBPs receive less attention than other contaminants. Many emerging contaminants – pharmaceuticals, for example – are rarely found in treated drinking water and, when they are, they are present at low ppt levels – and it’s the same story with perfluorinated compounds, which have hit the headlines recently. DBPs, on the other hand, are always there, usually at ppb levels (sometimes mid- to high ppb levels). And that means we all face constant chronic exposure – we’re drinking this stuff, every day, and we don’t even know. We are missing more than 50 percent of the halogenated organic material that is formed in chlorinated water, and we are probably missing much more in other disinfected waters. Right now, we only have surrogate measurements to quantify how much we’re capturing.
In short, it’s an unsolved problem. And that’s a great driver for me!
A healthy interest
Back when I first heard about DBPs in 1991, haloacetic acids weren’t even regulated (only the four trihalomethanes were); a couple of people in the 1980s had identified a few additional DBPs, but the work didn’t gain much traction. Then two scientists told me there were links between drinking water and bladder cancer. At that point, we didn’t even know about the risk of earlyterm miscarriage. Many people continue to believe that since DBPs are regulated by the Environmental Protection Agency in the US, the problem is solved. But I realized that none of the regulated DBPs cause bladder cancer in animals. Many scientists, me included, think that we are missing important compounds that are actually responsible for human health effects.
Clearly, I can only do so much towards solving a big health issue like this. I continue to collaborate with several EPA toxicologists and epidemiologists. As a chemist, I can’t know whether these things are toxic or not – though I have good intuition! My job? To keep identifying and quantifying to give us a handle on the problem. We can’t quantify everything – it’s too much work for a small group of people – but we have some good in vitro toxicology data on around 140 DBPs, and we are getting an idea of what features in the chemical structures are causing the toxicity, and which ones we need to hone in on. Ultimately, we want to try to remove them during drinking water treatment.
We’ve just started a new project with the state of Minnesota looking at the ecological impact of DBPs in rivers, mostly focused on chlorinated wastewater that enters the rivers. We don’t yet know about DBP input into the rivers, their levels or how they might affect aquatic life. We also have a study looking at treatment technologies for a whole suite of compounds that are very difficult to remove – 21 for this particular project – because they have been prioritized in California for potable reuse. A few cities are doing either indirect potable reuse, where they turn wastewater into drinking water, or more direct reuse. Many cities have what is called “de facto” reuse, where they are receiving treated wastewater from other cities upstream and treating that contaminated river water for drinking water. These contaminants can cause ecological problems with fish and other aquatic life. So, we’re combining human health and ecological health, and working with engineers to find better ways of eliminating those compounds.
Tools of the tox trade
Many DBPs are lower molecular weight – under 500 MW – so GC-MS tends to be the technique we turn to most often. It’s also easier to identify new compounds with GC-MS. We find highresolution accurate-mass MS valuable, as the rich dataset guides us to the molecular formula (although we still need to manually interpret the spectra). LC-MS can give us access to the highly polar compounds that GC can’t detect without derivatization techniques.
LC-MS is handy when you know what you’re looking for, but it can present challenges when you’re doing non-targeted, unknown analysis. Solutions (software tools in particular) are on the horizon, especially in the emerging contaminant world, and many people are developing workflows with an eye on automation. We also need improved databases and libraries. The NIST library has more than 200,000 molecules, but there are many more. I would say about a third of what we see in drinking water does not appear in the library. We created our own user library database, comprising newly identified DBPs that aren’t in the NIST library – and we’re detecting more all the time.
ORIGIN STORY
At graduate school, I was not focused on environmental research. I was not even studying analytical chemistry; I was doing physical/organic chemistry. But I had the opportunity to run the mass spectrometry lab in the chemistry department and gained some good experience using MS, which led me into learning how to identify unknowns in samples.
When I was close to graduating, the general chem labs professor at Emory University told me that I should apply to the EPA National Exposure lab in Athens, Georgia. There wasn’t an opening at the time, I just sent a cold resume, but it turned out they had purchased the same really advanced HR magnetic sector mass spectrometer that I had been operating – and were clueless on how to use it!
I didn’t know anything about environmental chemistry until I joined EPA. It was all on-the-job training. I had experience identifying unknowns using HRMS, but now I had to apply it to environmental problems. I went to scientific conferences, I read the literature and learnt as I went – and I loved it. I morphed into this role.
About two years into my time at EPA, two scientists came to us – one from EPA Cincinnati and one from the University of North Carolina, Chapel Hill. They were both doing research on DBPs and, knowing that we had expertise in identifying unknown chemicals in water, they asked us to collaborate with them on a couple of DBP projects. At that time, there were very few people looking at drinking water; almost everybody was doing only the regulated DBPs. I recognized that it was an important problem, and we decided to combine their extensive expertise in DBPs with my background in identifying unknowns
One instrumental aspect that could be improved – and is increasingly being improved – is the limit of detection. Manufacturers continue to create instruments that push the limits, and that’s helping a lot. One of my former postdocs is using a newer mass spec to develop quantitative methods for a suite of DBPs – without doing any extraction or preconcentration. Wouldn’t it be great if a mass spec worked like it does on forensics drama CSI? Simply inject the water sample, and receive the answer! In all seriousness though, we cannot extract everything – and we don’t know what we’re not extracting.
Another limitation is the availability of standards. When we identify new compounds, we want to confirm as many as we can if we think they’re important toxicologically but, often, we can’t purchase those standards. I’ve had well over 200 compounds synthesized by chemists in the lab so that we’re able to match some of the DBPs we’ve tentatively identified. When targeting certain DBPs and quantifying them with LC-MS, it’s best to use isotopically labeled standards to get around any matrix effects – and you could still miss something.
Unfortunately, such standards are very expensive (sometimes US$1,000 each!), so we can’t always afford them – and they don’t exist for a lot of compounds. If you’re doing unknown identification – even with HRAM-MS or MS-MS – until you confirm it with a chemical standard, it’s only a tentative identification (even if you’re 99 percent sure). And I’ll admit, we have been wrong in the past. In short, I’d say that DBP analysis is complicated!
Carbon trading
My current project on granular activated carbon (GAC) is super exciting. It’s a similar principle to a BRITA water filter, which uses activated carbon to remove chemicals, such as lead, bisphenol A and so on, from drinking water. On a larger scale, GAC can be used at water plants to remove the precursors to DBPs – the natural organic matter and various anthropogenic contaminants.
In the past, people said GAC was too expensive, and that we could only use it when we had dire need. I’d argue we’re at that point! Why? Many cities have switched from chlorination to chloramination to lower the concentrations of regulated DBP levels; in other words, instead of removing the precursors, they’ve just changed the treatment method. However, we’re now finding out the negative side of chloramination – including iodinated DBP formation. Even the EPA is rethinking whether GAC is a better way to go. If we can remove the precursors at the very beginning, we can prevent DBPs being formed.
We’re collaborating with a couple of engineering research groups, such as NC State, University of Colorado, and Hazen and Sawyer, and looking at pilot plants and full-scale plants around the country. We’ve also done some controlled lab experiments with miniature GAC columns. We’ve seen promising results – up to an 80 percent reduction in many DBPs with “young” GAC.
In particular, we’ve focused on the lifespan of the GAC. Just like your BRITA filter, when a GAC filter is brand new, it’s going to do the best job (80 percent reduction in DBPs as mentioned above) but, as it ages, it becomes less effective. In “middle age,” we see around a 50 percent reduction in DBPs. But, promisingly, we found that old GAC systems still deliver a significant reduction in DBP formation after many months of use.
We did find a few “gotchas.” For example, at a plant with no GAC filter – just chlorinated water – we don’t see any tribromonitromethane (a really toxic DBP); but, after adding GAC, it starts forming. In other words, GAC can, rather ironically, cause the formation of some toxic DBPs. When it comes to brominated DBPs, it’s because the GAC removes organic matter, but does not remove any bromide, which changes the ratio of organic matter to bromide.
So does GAC make drinking water safer? We did a calculated “TIC tox”approach whereby we multiply the concentration of both regulated and unregulated DBPs by the toxic potency. What that doesn’t tell you is the total toxicity of the whole drinking water mixture, which includes compounds we aren’t measuring or don’t even know are there. That caveat notwithstanding, the use of chlorination with GAC resulted in substantial reductions in the calculated toxicity compared with water not treated with GAC.
Going forward, giving back
When I first got into this area, people were only measuring regulated DBPs, most of which are not as toxic as the new unregulated ones we have identified – but we helped trigger a whole area of research. We have assembled the right multidisciplinary people – toxicologists, engineers, epidemiologists, chemists, regulators – and have the expertise to help solve this problem.
The more people are involved, the faster we can solve problems. I may not be able to do it with my own two hands and my own circle of collaborators, but there is now a larger group of people who recognize the importance of DBPs, and are prepared to work on new compounds they’ve discovered. When I started here at USC in Jan 2014, I had an all-woman team of grad students (and one undergrad student) who helped with our research. These students helped me create extremely sensitive analytical methods which allowed us to quantify these very toxic DBPs at the low levels we need and I’m so proud of them. In a government setting, there is lots of money for instruments and supplies, but no money for people. In universities, we have the reverse problem: no money, but great students in the lab all as enthusiastic as I am – sometimes even more so! Without them, we wouldn’t be at this point now. That keeps me motivated.
We now know a lot more than we did about DBPs and their impact (though there is much more we need to know!) and we’re getting close to finding some good solutions. Many scientists are happy to publish what I call “pretty papers” that get cited – and I like that too. But what’s great is that I can do that as well as helping to solve an important human health issue and giving back to society. This research can ultimately help improve the lives of many, many people.
Arthur Sease Williams Professor of Chemistry at the University of South Carolina, Columbia, South Carolina, USA.