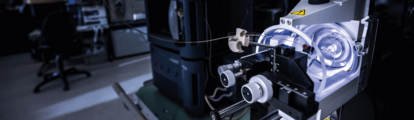
The Call of Coronal Duty
An insider’s perspective on the proteomic battlegrounds of COVID-19
Lauren Robertson, Perdita Barran, Jeroen Demmers, Manfred Wuhrer | | Longer Read
With March 11, 2021 marking one year since COVID-19 was officially classified as a pandemic by the WHO, it goes without saying that many of us – not least those on the frontline of the fight – are feeling a bit war weary. And yet, for so many in the analytical chemistry community who answered the call of duty back in 2020, the battle rages on.
With so much uncharted ground still to cover when it comes to this novel coronavirus and our response to it, there is an abundance of work ongoing across all sub disciplines of our field. For now, we decided to share some of the spoils from one battlefront in particular: proteomics. Here, experts Jeroen Demmers, Perdita Barran, and Manfred Wuhrer tell us about their work in the fight against COVID-19, and provide an insider’s perspective on some of the developments we can expect to see in the coming months.
How can proteomics help in the fight against COVID-19?
Understanding the role that proteins play in the SARS-CoV-2 infection process and disease progression is vital to the development of therapeutic and preventative strategies. In this way, proteomics has proven to be an indispensable tool in COVID-19 research, and its role will no doubt be expanded in the future.
Firstly, MS-based detection of SARS-CoV-2 proteins and their proteolytic peptides offers a simple and rapid virus detection assay. Using targeted proteomics, peptides of the SARS-CoV-2 nucleocapsid and spike proteins can be detected with high sensitivity and specificity in research samples and clinical specimens. This opens up the possibility of taking this technology to clinical diagnostic labs and turning it into point-of-care procedures as alternatives for nucleic acid-based methods, which could be particularly interesting from a cost-effective healthcare perspective.
Proteomics could also be used to develop approaches capable of predicting COVID-19 cases that might later progress into clinically severe disease. In fact, several studies have already identified potential protein biomarkers that are differentially expressed in COVID-19 patients and could be used to predict viral infection at early stages. (See the sidebar “Collaboration and Determination” to learn more about Perdita Barran’s work around targeted proteomics and biomarkers).
In other areas, investigation of the humoral antibody response to SARS-CoV-2 proteins has aided the development of antibody-based assays for diagnostic and therapeutic purposes. Recently, a comprehensive SARS-CoV-2 human protein–protein interaction map was generated using affinity-purification (AP) MS. Several hundreds of specific interactions between SARS-CoV-2 and host cell proteins were defined, and it was discovered that, for some of the involved human proteins, several existing FDA approved drugs were already available. Other proteomics-based research on the host cell response has shown that the complement system and metabolic pathways are severely affected in COVID-19 patients.
Unbiased, explorative proteomics has also been used to define the proteomes of autopsy samples from COVID-19 patients. For instance, it was shown that cathepsin L1, rather than ACE2, was significantly upregulated in the lungs of COVID-19 patients. In addition, systemic hyperinflammation and dysregulation of glucose and fatty acid metabolism was detected in multiple organs, which shows how the multi-organ proteomic landscape of such autopsies may help in our understanding of the biological basis of COVID-19 pathology.
Crosslinking MS has been used to study the interaction sites between antibodies and the spike protein in detail. Such studies, often combined with protein structure elucidation by tools such as cryo-EM are crucial in the development of antiviral therapeutics. In research studies, huge non-covalent assemblies of proteins – such as intact virus particles several 10s of Megadaltons in mass – can be analyzed by MS. This way, conformational dynamics of viruses and viral proteins can be uncovered and this can yield valuable information on the stability and topology of macromolecular assemblies in general and virus capsid structure in particular.
Viral proteins, in particular those in the viral envelope (such as the spike protein), are extensively decorated by protein glycosylation. To understand how this post translational modification influences spike-ACE2 interactions with the host cell membrane, these glycan structures have been characterized in detail by (glyco)proteomics. Detailed analyses of the impact of emerging variants in spike and natural or designed-for-biologics variants of ACE2 on glycosylation and binding properties are important next steps in developing therapeutics.
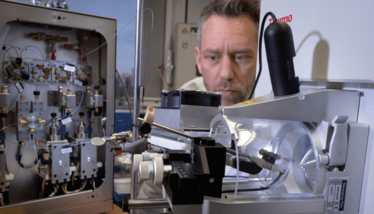
COVID-19 Detection: Hitting the Mark
How we successfully used targeted proteomics for the detection of SARS-CoV-2 proteins
By Jeroen Demmers, Director of the Proteomics Core Facility and Associate Professor of Proteomics, Erasmus University Medical Centre, the Netherlands
Early last year, once it became clear that COVID-19 had started to spread out across the world, there was a general sentiment in Europe that it wouldn’t happen that easily here. Just like the SARS and MERS coronavirus pandemics that came before (in 2003 and 2013, respectively), many people thought that this novel virus would be kept out of the region as well. Soon enough, the pandemic hit northern Italy hard, and it wasn’t long before there were messages of infected people in the southern province of Noord-Brabant in the Netherlands.
The first official outbreaks were reported in early March, and things developed quickly from there. In the second half of March our institute was shut down – like many others across Europe – and only research on COVID-19 was allowed to continue. For us, this work was happening at the Viroscience department at Erasmus MC, where several research groups had been focusing on coronaviruses for decades. My research lab and core facility had a choice: shut the lab, or grab this opportunity to contribute to SARS-CoV-2 containment by adapting our technology for use in virus detection and – if successful – diagnostics.
The journey to discovery
Thanks to our previous work during the MERS coronavirus pandemic (our lab had identified the MERS-CoV human receptor protein using MS-based proteomics; see “Gone Fishing” for more information), we had already established connections with the Viroscience department. I decided to contact coronavirus specialists Bart Haagmans and Mart Lamers as I knew they were working day and night on SARS-CoV-2 assays to answer questions about the mechanism of infection. For one of their assays, they were interested in analyzing the response of the host cell proteome to viral infection in a recently developed organoid-derived bronchioalveolar tissue culture. Using our technology, we were able to help them monitor up- and downregulation of large numbers of proteins upon viral infection and learn more about the intracellular pathways that are turned on or off as a result of infection. Because of this work, and our connections with the Viroscience department, we were also granted access to some of their interesting SARS-CoV-2 samples – meaning we could test whether it was possible to measure viral proteins in complex samples, such as cell lysates.
We started off with samples from an infected Vero E6 cell line derived from the African green monkey – this cell line is used to propagate viruses and serves as a rich source of viral material. A dilution series was then created to demonstrate the limit of detection of specific viral proteins. As the virus was already genotyped, the protein amino acid sequences that we needed for the analysis of proteins based on peptide fragmentation or MS/MS data were already available. Also, we were quite lucky (or unlucky?) in that just a few days before most of the institute was shut down, a brand new Orbitrap Eclipse MS was installed in the lab. The first proteins analyzed on that machine were SARS-CoV-2 proteins!
The output of a standard proteomics experiment is usually a table of identified proteins, which is generated in the final step of a database search using software tools that may take up to several hours. The progression of this process (at least in the tool that we use) is indicated by a green bar. I clearly remember the anxiety and excitement that we felt when, after the very first database search, we saw the bar hit 100 percent and the list of identified proteins popped up: the first time we identified SARS-CoV-2 proteins really felt like looking the monster directly in the eye.
Gone Fishing
For our work on the MERS-CoV human receptor protein, we designed a relatively simple "fiishing" experiment that was performed using in vitro synthesized MERS-CoV Spike 1 protein immobilized on magnetics beads. The spike protein was used as bait, and we went fishing in a pond of human proteins – or "cell lysates," prepared by crushing cells that were cultured in a petri dish in the presence of detergents. We found one human protein that showed a very specific interaction with the bait protein, suggesting this was a receptor protein present on the outside of the human host cell – for example an epithelial cell in the lung that the virus grabs and uses to enter the host cell.
The identification of the receptor protein was not only crucial in understanding how the virus infects a host cell, but also for the development of antiviral therapeutics and vaccines. For instance, by blocking a receptor using small molecule drugs or antibodies, infection of the host cell can be prevented. We identified the protein DPP4 as the receptor for MERS-CoV, and this finding was confirmed by in vitro and in vivo experiments (1). Since it is different from the ACE2 receptor that SARS uses to enter the host cell, this was a somewhat unexpected finding at the time.
From a proteomics point of view, this study is the ultimate example of the importance of identification of proteins in an unbiased manner – the core of MS-based proteomics technology. If more conventional experimental methods, such as screening assays, had been used at that time, the identity of the receptor is unlikely to have been found so quickly. One would have to have made a selection of possible receptors a priori, and if the protein was not included in that selection, it would not have been found.
The right sample
For a few months, we worked on nothing but COVID-19. Virtually all other projects were put on hold and since most meetings at work were cancelled and there were no teaching duties, it really felt like a postdoc project where the full focus is on basic science. I truly relished this lack of distraction, despite the troubling situation we – as citizens of the world – were in. On a personal level, we were building a new house and we weren’t sure whether we could still sell our old house – what with the threats of a housing market collapse together with the crashing stock markets and other doomsday scenarios that circulated. It was truly both an exciting and troubling time.
We soon identified a set of proteolytic peptides that could serve as the target peptides in follow-up experiments. Also, we were able to calculate limits of detection for viral proteins in our proteomics assays. Under ideal conditions, we could go down to the mid- to low attomole range in targeted experiments, just like the numbers we had seen before in another project on non-related proteins.
While setting up these assays, I had already started asking around for patient material to see whether we could detect proteins in clinical specimens (such as nasal swabs) and to determine if it could be used as a diagnostic tool. However, getting patient samples turned out to be more difficult than I had anticipated. For conventional PCR-based testing, samples are usually stored in a "transport medium." This medium contains a lot of protein, the signals of which dramatically mask the signals of viral proteins in our assay. Unfortunately, adaptation of standard protocols in diagnostic departments is virtually impossible, and as research scientists who are used to changing protocols if something doesn’t work, this was quite frustrating.
But one day we got a message from a collaborating clinical virologist who had collected a different type of sample from a COVID-19 patient. This was a sputum sample, deposited on a little glass slide with no addition of transport medium or any other buffer solution. Upon inactivation of the virus in 80 percent acetone, we could take the sample from the BSL lab to our own lab and subject it to our standard bottom-up proteomics protocols – which basically means digesting all the proteins into peptides. This sample was in fact the first clinical specimen in which we could clearly detect SARS-CoV-2 peptides.
We used a targeted proteomics assay, which means that we set the MS in such a way that it only detects viral peptides that were selected a priori. The quadrupole in the Orbitrap hybrid MS then acts as a filter that lets only the peptides (or m/z values) of interest pass through. Upon fragmentation of the peptide to determine the amino acid sequence, the fragment ions are measured in the Orbitrap with high selectivity and sensitivity – the high mass accuracy of the Orbitrap is a clear advantage over such targeted methods in a triple quadrupole instrument here. The fragment ion fingerprint that is thus obtained is highly specific for the selected peptide. These fingerprints are then computationally compared with the specific fingerprints that were defined in the experiments on infected Vero E6 cells. Using targeted MS, the sensitivity can be increased and the limit of detection is at least 10-fold lower compared with data-dependent acquisition (untargeted) MS.
Next, we contacted clinical virologists from a hospital in the south of the Netherlands, which was located in the center of the area that was hit by the first COVID-19 wave in the spring of 2020. The clinicians there used so-called Eswabs, for which no protein rich transport medium is necessary. This results in much less background in our analyses and therefore increased sensitivity. Despite the excess of red tape, we managed to get an Eswab sample cohort to our lab. This sample set contained various swabs within a wide range of PCR Ct values and we could see a nice inverse correlation between Ct value and peak intensities of target peptides in the mass spectra, reflecting the abundance of proteins. Later, in a second sample cohort, we managed to get similar results and could detect SARS-CoV-2 peptides at fairly high Ct values (i.e. low viral counts).
Winning the war…
Where are we now? We have established the proof-of-concept and have shown that it is definitely possible to detect SARS-CoV-2 proteins using MS. The challenge now is to translate this methodology from the R&D stage to the clinical diagnostic lab. For the analyses we have performed so far, we used state-of-the-art, ultra-sensitive Orbitrap mass spectrometers – which are typically not present in clinical diagnostic labs. Still, the basic technology is comparable to triple quad MS and these are readily available in many clinical labs.
There is still a debate around the level of sensitivity we really need in COVID-19 testing. The limit of detection of PCR based methods is unsurpassed, but do we really need that sensitivity? It is unclear whether infected individuals, whose nasal swab PCR Ct values are in the high 20s or low 30s, are infectious. Although no viral proteins could be detected in most swabs with associated Ct values of >26, we have to test whether the sensitivity that can be reached by MS-based approaches is sufficient to differentiate between infectious and non-infectious people. Only then will we be able to assess the potential value of MS-based COVID-19 testing.
One clear advantage of this technology over other testing methods however, is that proteins of multiple different viruses can be targeted in one assay. If peptide signatures for a given virus are defined, these can be relatively easily included in the target list. This way, samples can be screened for multiple viruses simultaneously. This is not only useful now, but also in the future when differentiation between different pathogens will be needed.
One challenge to overcome will be improving the analysis time: the sample preparation for proteomics assays takes a while, mainly because of the protein digestion step. This can be dramatically reduced by microwave irradiation. Furthermore, LC gradients could be much shorter than they are now: we have managed to reduce the gradients lengths threefold and could still detect most of the SARS-CoV-2 peptides. Running clinical samples using LC gradients of only up to a few minutes should be possible.
As a final note, I’d like to mention that part of our early work was published on biorXiv. Although manuscripts are not peer reviewed, they can be downloaded by the scientific community and the general public for free. For COVID-19 research, this has been a tremendous help in the dissemination of data, knowledge and protocols. Even though our manuscript has not yet been published in a scientific journal, our selection of target peptides and MS data sets have been used by others and already proven useful. I believe this is a beautiful illustration of the power of open access scientific knowledge – a trend I hope to see continue in the future.
I’ve demonstrated my own work using MS to detect viral proteins, but this is just one application of this versatile technology. It is clear to me that by studying proteins, both from SARS-CoV-2 and the human host cell, proteomics has profoundly changed the way we study viral infection and disease progression at the molecular level. I am excited to see the many potential novel applications that will no doubt come to fruition in this fast moving field.
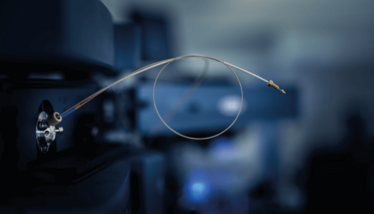
Collaboration and Determination
Perdita Barran, Professor of Mass Spectrometry at the University of Manchester, UK, shares a targeted approach to SARS-CoV-2 proteomics
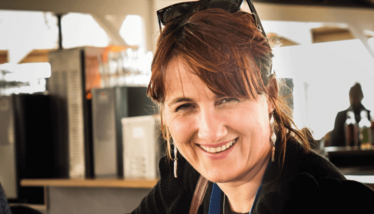
How can targeted proteomics help in the fight against COVID-19?
Targeted proteomics can help diagnose whether someone has the virus, but it can also help to determine the effect of the virus on a given individual, by providing biomarkers that can predict the course of the disease. I am working on projects in both of these areas. Ultimately, targeted proteomics (and indeed metabolomics and lipidomics) could provide the cheapest and most robust methods to determine the course of infection – and to help doctors decide how to treat individuals.
Can you tell us a bit more about your own work?
We have found that the NCAP protein in SARS-CoV-2 is very amenable to fast digestion, and that it can be detected at 100 attomol level by UPLC-MS. This means we can determine how much viral protein is present in any individual sample. MS directly measures the viral protein, without any labeling or the need for many additional reagents.
What is interesting to me in this area at the moment is comparing the abundance of viral protein (as found by MS) to viral RNA (as detected by RT-PCR). It may be that the viral protein abundance is a better indication of infectivity, as there are lots of reports of RNA hanging around much longer than an individual is infectious. Maarten Dhaenens has been one of the pioneers in translating a method for clinical diagnosis of COVID 19 with MS.
The other role for targeted MS will be to determine the presence of mutations in the virus. This diagnostic capacity could be extremely helpful in surveillance testing, as we will need to know if the vaccine continues to provide immunity – especially as new strains emerge.
How has MS added value to the pandemic so far – and what about its future impact?
To date there are 244 published papers on PubMed that have COVID-19 and MS in the title or abstract. This is likely an under representation of the role that MS has played in this pandemic. Increasingly, scientists are using MS to study the progression of the disease with renewed focus on understanding the effects of long COVID. I think MS will have an important role in the development of therapeutics to treat people with the virus, as well as contributing to vaccine development.
More importantly, the fact that so many scientists have been willing to work together and share knowledge has been incredible. The COVID-19 MS coalition, which I helped initiate, is a great example of this – within a few weeks we had more than 800 members. Actions like this, in the face of the threat of the virus on all of us, will lead to more collaborative science and allow us to develop public health that is less competitive.
I also hope that any new resource being purchased for coronavirus research will benefit the diagnosis and treatment of other diseases. The data being collected now all over the world will be a great future resource. The way we’re accelerating rapid diagnostic tests to the point of having them validated and being used by clinicians is a real celebration.
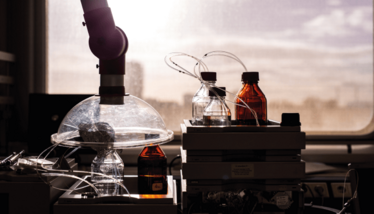
A structural and systems biology view
Manfred Wuhrer, Professor of Proteomics and Glycomics at Leiden University and Head of the Center for Proteomics and Metabolomics in the Netherlands, shares his view on how MS-based proteomics can contribute to COVID-19 research in the clinical lab
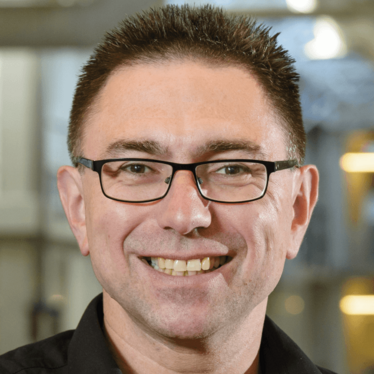
What’s the role of MS in structural research around SARS-CoV-2?
MS largely contributes to the structure elucidation of the spike protein. Initial bottom-up proteomics studies showed that the spike protein is heavily glycosylated. This did not come as a surprise, as SARS-CoV-2 shares this feature with many other viral surface glycoproteins – it was remarkable how quickly different laboratories then performed in-depth analyses of the glycosylation of the S protein! These studies provided key insights into how glycans shape the viral surface and influence the interactions with host cell factors and the immune system.
Do you think MS could make a useful (and realistic) diagnostic tool for COVID-19?
Current COVID-19 molecular diagnostic assays focus on the detection of parts of the viral genome (PCR-test) or protein antigens (“quick” or even “self-test”). At the moment, MS does not play a role in diagnostics, but it certainly has a lot of potential in this direction. Various efforts are ongoing to establish assays for the low-resolution MS detection of viral proteins to obtain a molecular fingerprint of diagnostic value from, for example, nasal swabs or even gargle solution. These attempts build on the previous success of whole-cell MS and intact mass analysis of major microbial proteins – something that is now widely used for diagnosing bacterial and fungal infections. I am curious to see whether the MALDI-TOF-MS platforms that are widely established in medical microbiology laboratories will find their way into the diagnosis of viral infections, including SARS-CoV-2.
The bottom-up approach chosen by Jeroen Demmers likewise has good potential for translation into clinical laboratories. After transfer of these assays onto triple quadrupole LC-MS platforms, they can certainly be established in clinical chemistry laboratories, which often have the necessary hardware and increasingly also the protein expertise available.
What about the use of biomarkers in clinical diagnostics?
COVID-19 often has a huge, systemic impact on infected people, and there’s a range of immunological, cell biological, and metabolic effects with biomarker potential. Due to the enormous impact of the pandemic, an array of omics technologies have been applied to COVID-19 patient materials which has led to a range of promising biomarkers. Using MS, my team has recently helped to define a specific glycosylation switch on antibodies against the viral spike protein in COVID-19 (2). This switch appears to initiate inflammation, and we are now looking at whether it can serve as an early marker predicting the development of severe COVID-19. I think a key challenge will be to integrate and scrutinize this wealth of information using a meaningful, systems biology and systems medicine approach.
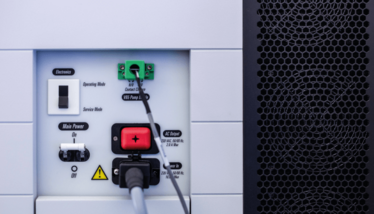
- VS Raj et al., Nature, 495, 251 (2013). DOI: 10.1038/nature12005
- M Larsen et al., Science, eabc8378 (2020). DOI: 10.1126/science.abc8378
By the time I finished my degree in Microbiology I had come to one conclusion – I did not want to work in a lab. Instead, I decided to move to the south of Spain to teach English. After two brilliant years, I realized that I missed science, and what I really enjoyed was communicating scientific ideas – whether that be to four-year-olds or mature professionals. On returning to England I landed a role in science writing and found it combined my passions perfectly. Now at Texere, I get to hone these skills every day by writing about the latest research in an exciting, creative way.