
The Great Sewage Census
From revulsion to revolution: wastewater analysis not only informs us of human health and habits, but could even help transform our approach to healthcare.
Rolf Halden |
The first thing people ask when they hear that we analyze wastewater: “Why on earth would you pick that as your subject?” And, while the question is understandable, the answer is simple: to monitor health and protect the general public.
I was not the first to hit on the idea of studying sewage; people have monitored microbes in wastewater to study epidemics as far back as the 1950s, and European researchers have measured levels of illicit drugs across multiple cities since the early 2000s. Our primary contribution to this area has been expanding the study of wastewater into a platform for evaluating matters of public health. My story, however, did not start with sewage. It started with something even less pleasant…
Protecting the public
My first job after completing my doctorate was as an environmental engineer at Lawrence Livermore National Laboratory – a weapons lab – where I dealt with hazardous chemicals, such as organochlorines, dioxins and polychlorinated biphenyls. It was five years later, in 2001, that I moved to Johns Hopkins University to start up the “Center for Water and Health” program as an assistant professor and found myself surrounded by public health experts – it is the biggest school of public health in the world, after all. I hoped that my knowledge of organochlorines (persistent molecules incompatible with natural degradation systems) and dioxins (polychlorinated carcinogens consisting of two aromatic rings) would be of use in this new environment. It was, and I quickly identified not just one but two chemicals to study in wastewater – triclosan and triclocarban, both antimicrobials.
The significance of triclosan (a trichlorinated sanitary agent) in the public health sphere was obvious. Not only was it recognized as a pre-dioxin by the US Environmental Protection Agency (EPA), meaning it can serve as a precursor to more persistent and increasingly chlorinated dioxins during incineration, but it could also contribute to the development of microbial drug resistance. Coupled with the fact that it was present in many personal care products at the time, triclosan represented a promising research target and many teams had already set out to study its relevance. I saw an opportunity to bring a new dimension to our understanding using wastewater analysis.
The second antimicrobial that piqued my interest, triclocarban, also has a persistent structure, comprising two aromatic rings and three chlorines, and was in widespread use at the time. And yet, it hadn’t been studied at anywhere near the level of triclosan. Why not? Because triclosan could be studied by GC-MS while triclocarban could not – it reacted with the column and wouldn’t make it to the detector. To make matters worse, sample derivatization to improve the analysis was also difficult. Luckily, I like a challenge!
Given the lack of information available, I chose to focus on triclocarban first, with a view to answering two fundamental questions: i) what methods could we use to study this compound, and ii) where does triclocarban end up after its use in hand soap?
To address the first question, we adopted LC-MS and LC-MS/MS. LC-MS was fine for measurements in simple environmental samples (ground water and drinking water, for example) (1). Initially, the triclocarban eluted with another compound of the same molecular weight, but the addition of acetic acid led to the formation of a triclocarban adduct whose heavier reference ion moved it away from the interference, providing a much clearer result. In time, as we moved on to more complex samples, like sewage sludge or human materials, our team also started to use a tandem MS method we developed (2).
When it came to finding triclocarban, I knew that it was formulated into soap – and what happens to soap? It is used and washed down the drains into wastewater. From our lab in Baltimore, we followed wastewater pipes to the nearest treatment plant and found triclocarban not only in the water entering the plant, but also in urban streams near leaky sewer pipes and in the process flows exiting the plant – both the reclaimed “cleaned” water and in the “sewage sludge” produced as a byproduct of water reclamation. Next, we studied additional urban streams passing through Baltimore (and representing potential drinking water sources) as well as brackish waters of nearby Chesapeake Bay and also of Jamaica Bay near New York; we found triclocarban at all of these sites, including in the sediments of Chesapeake and Jamaica Bay. At that point, we decided to look at triclosan, and found that it was also present in all of the samples.
Double trouble
From a public health perspective, the co-occurrence of the two drugs spelled trouble but it provided a benefit in terms of predictive power. We developed a simple model forecasting the concentrations of triclocarban based on triclosan levels detected across the nation – our model predicted that triclocarban was likely a top 10 contaminant in drinking water across the US (3). The prediction was largely based on data from the EPA and the US Geological Survey, who had measured triclosan in numerous locations, including in sewage sludge and freshwater streams. Of course, when we published our paper, we came under pressure to prove our estimates… And so that’s what we did.
I spoke to the EPA in the hope of expanding our wastewater data and, luckily, they had just completed their nationwide sewage sludge survey. They had recovered samples of sewage sludge from all over the US, stored them, and were preparing to dispose of them (they had reached the end of their four-week shelf life). I rushed over there with a bunch of coolers to take as many as I could off their hands; I figured that any organic chemicals remaining in the sludge at this point must be highly resistant to degradation and would likely be of anthropogenic origin – and therefore important to my study. Using the EPA samples, we were able to produce national inventories demonstrating the total mass of triclosan and triclocarban in wastewater across the US, quickly expanding to over 200 wastewater treatment sites across the nation.
Armed with data on of the widespread co-occurrence of these compounds and their persistence after wastewater treatment – a process that should destroy anything degradable – our next priority was to investigate the potential for people being exposed to these chemicals, which could result from drinking contaminated water or the consumption of agricultural products from land that has been treated with sewage sludge – over 14 billion pounds of sludge are produced each year in the US, and approximately half of this is spread on land. Perhaps unsurprisingly, we found the two antimicrobial compounds pretty much everywhere we looked for them – in breast milk, in umbilical cord blood and in the urine of young children, to name a few examples.
Though not everyone is concerned for the environment, most do take great interest in their own health and the health of their families. Accordingly, our findings in humans triggered a cascade of new research. The link between chlorinated aromatic compounds and endocrine disruption was already known, but as more and more researchers began to look at the health effects of these chemicals, the list of their harmful effects mushroomed. When you consider the entire lifecycle of these compounds, the list grows even more damning (4); for instance, triclosan manufacture generates a carcinogenic dioxin byproduct and triclocarban degradation gives rise to further carcinogens. It became very clear that these chemicals posed a risk to public health.
The Human Health Observatory
We soon began using the wastewater as a diagnostic matrix to study much more than exposure to just one or two select contaminants. First, we added antibiotics to our hit list, because of concerns surrounding the rise of antibiotic resistance, and found extremely high concentrations of these compounds in sewage sludge. Soon, other chemicals relevant to public health got added to the list – illicit drugs, prescription drugs, stress hormones, antidepressants, and nicotine. Before long, our panel of analytes had expanded from two chemicals and their transformation products – maybe a dozen in all – to several hundred different compounds that we investigated in raw and treated wastewater as well as in sewage sludge.
Our work was no longer limited to the US, either. To date, we have conducted measurements at over 100 locations in China alone and probably well over 350 locations around the world in total, meaning that our method has been used to estimate the behavior and health status of an estimated 200 million people worldwide. This data and the corresponding global sample archive, dubbed the “Human Health Observatory”, is collected at relatively low cost and gives us the power to manage health in a preventative manner, rather than reacting to observed outcomes. In other words, we can measure threats before they make people sick – not only chemicals but also biological agents, such as viruses and antibiotic resistance genes. Communities can then take the appropriate precautions against the identified threat; for example, equipping emergency responders with an appropriate drug or antidote.
Such proactive healthcare management is an important goal. The US alone spends three trillion dollars a year on healthcare, yet we still focus most of our efforts on curing sick people rather than on preventing the healthy from becoming sick in the first place – it’s like cleaning up your flooded bathroom without first shutting off the valve to the broken pipe. We have already demonstrated that wastewater analysis can flag the arrival of a new dangerous drug or disease to a neighborhood or area (5) (6), and we have outlined how this approach can be used to avoid epidemics and pandemics in the future (7).
In the case of persistent molecules like dioxins and polychlorinated biphenyls – which are lipophilic and accumulate in adipose tissue – another bonus is the ability to avoid invasive biopsy procedures. In fact, we published a paper in Nature Scientific Reports showing a tight correlation between the levels of these chemicals in sewage sludge persisting after treatment and in human adipose tissue, essentially allowing the estimation of the types and magnitude of toxic burdens in populations based on measurements made at the wastewater treatment plant serving these exposed populations (8).
At a more local level, we contribute to proactive healthcare efforts in our home city (Tempe, Arizona) by talking to the mayor’s office on a monthly basis about threats we identify in the wastewater of our community, and we also work closely with the federal government. I have presented to the US EPA on multiple occasions regarding the inventories and trajectories of persistent chemicals and these sessions opened up discussion with the CDC and FDA, with the latter agency eventually making the decision to ban triclosan, triclocarban and numerous other antimicrobials from products under their supervision. That marked the beginning of the end of overuse of risky antimicrobials in personal care products, and it is a rewarding feeling that our team played a part in it.
Ultimately, when I think about the journey my research has taken me on, I’m reminded of a quote from the philosopher Arthur Schopenhauer:
“All truth passes through three stages. First, it is ridiculed. Second, it is violently opposed. Third, it is accepted as being self-evident.”
We have certainly seen ridicule (“Why would you search for old samples that are past their shelf life?”) and have heard plenty of criticism (“You’ll never know which individuals are represented in the samples!”). In fact, I left Johns Hopkins back in 2008 to join the Biodesign Institute at Arizona State University because federal funding – on which Hopkins relied – was so hard to obtain for this unconventional work. But our results speak for themselves, many critics now recognize the benefits of the approaches we use, and we now have the satisfaction of seeing our work make a real difference in the world.
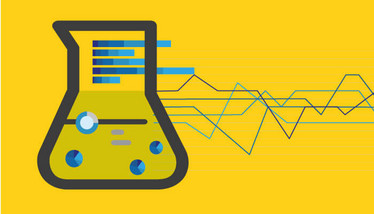
What can we learn from these enormous databases that track community behaviors? We delve into one example, where researchers in the city of Tempe, Arizona, are tracking opioid use in real time...
- RU Halden, DH Paull, “Analysis of triclocarban in aquatic samples by liquid chromatography electrospray ionization mass spectrometry”, Environ Sci Technol, 38, 4849 (2004). DOI: 10.1021/es049524f
- A Sapkota et al., “Detection of triclocarban and two co-contaminating chlorocarbanilides in US aquatic environments using isotope dilution liquid chromatography tandem mass spectrometry”, Environ Res, 103, 21 (2007). DOI: 10.1016/j.envres.2006.03.006
- RU Halden, DH Paull, “Co-occurrence of triclocarban and triclosan in U.S. water resources”, Environ Sci Technol, 39, 1420 (2005). DOI: 10.1021/es049071e
- RU Halden, “On the need and speed of regulating triclosan and triclocarban in the United States”, Environ Sci Technol, 48, 3603 (2014). DOI: 10.1021/es500495p
- AJ Gushgari et al, “Tracking narcotics consumption at a Southwestern U.S. university campus by wastewater-based epidemiology”, J Hazard Mater, 359, 437 (2018). DOI: 10.1016/j.jhazmat.2018.07.073.
- AJ Gushgari et al, “Long-term tracking of opioid consumption in two United States cities using wastewater-based epidemiology approach”, Water Res, 161, 171 (2019). DOI: 10.1016/j.watres.2019.06.003.
- R Halden et al., “Tracking harmful chemicals and pathogens using the Human Health Observatory at ASU”, 11, 1 (2019). DOI: 10.5210/ojphi.v11i1:9843
- AK Venkatesan, RU Halden, “Wastewater treatment plants as chemical observatories to forecast ecological and human health risks of manmade chemicals”, Sci Rep, 4, 3731 (2014). DOI: 10.1038/srep03731
Founding Director of the Biodesign Center for Environmental Health Engineering at the Biodesign Institute, Professor in the Ira A. Fulton School for Sustainable Engineering and the Built Environment, and senior sustainability scientist in the Global Institute of Sustainability at Arizona State University, Tempe, USA.