I grew up wanting to be a surgeon, a laudable ambition with one fatal flaw – I am extremely squeamish. Luckily, I loved physics just as much as biology. Completing my PhD here at the UK’s National Physical Laboratory (NPL) taught me just how important measurement science is – and sparked my fascination with mass spectrometry imaging (MSI). Over the next 20 years, I developed a world class capability in secondary ion mass spectrometry (SIMS). Most of our work was in devices, organic semiconductors, and advanced manufacturing, but I could see huge potential for these techniques in biology.
Five years ago, I established the National Centre of Excellence in Mass Spectrometry Imaging (NiCE-MSI), with the goal of bringing physical metrology to the life sciences. Now, a large amount of my research is with the pharma industry, trying to better understand how drugs interact with cells, and so reduce drug attrition. The Centre started with just five people, and it’s now grown to one of the biggest MSI centers worldwide, with 22 staff and 20 PhD students.
In vino veritas
These days, my ultimate research goal is to achieve “super-resolution metabolic imaging”. Super-resolution microscopy has been absolutely transformational for the life sciences; it lets us peer into the machinery of life – the proteins that make up our cells. With MSI, I believe we can do the same with metabolites and drugs. It’s a big challenge. Unlike microscopy, MSI is typically label-free – labels can interfere with drug dynamics, and would be impossible for metabolites, which are constantly changing. There are other problems, too; SIMS allows us to focus down into the super-resolution space (under 250 nm). However, the mass spectra we get are of poor quality, so we cannot always identify the molecules accurately. One evening in May, 2011 I was enjoying a glass of Pinot Noir, while preparing a presentation for a forthcoming conference. My presentation discussed why the spectra we get from SIMS are so complex and hard to interpret. Largely, it’s because the MS instruments we use prioritize speed over accuracy. Speed is crucial to cover the millions of pixels needed for 3D imaging, but to do any serious work in life sciences, we need accuracy too. Unfortunately, the constraints of physics mean that it’s not possible to combine speed and accuracy into a single analysis – all MS is a compromise. To illustrate my point, I plotted a graph with speed on one axis, and accuracy on the other. I placed different mass spectrometer designs on this chart, from the super-fast time of flight (TOF) analyzers often used in SIMS, to the much slower but more accurate Fourier-transform mass spectrometers, such as the Orbitrap. As I looked at my chart, I had an idea – why not combine two mass spectrometers, one from each end of the spectrum, and so get the best qualities of both? At NPL, we take a lot of time and trouble to understand the measurement principles we’re working with; it allows us to see the big picture and spot gaps – and opportunities. It was clear to me that a hybrid instrument was the only way to get the qualities we needed, so that’s what we set out to create. The result was OrbiSIMS, which combines TOF and Orbitrap mass spectrometers. Orbitrap is well known in the life sciences for its high mass accuracy and mass resolving power, allowing us to find the smallest saplings within a forest of peaks. However, it is too slow for the 3D imaging we want to do; TOF-MS provides the speed. The combination of the two confers some important advantages, some of which only became apparent during development. It’s a little like a hybrid car – the fusion of a petrol engine and electric power gives a combination of qualities that would otherwise be unachievable, like rapid acceleration and fuel efficiency.Better together
For readers who are not familiar with SIMS, it involves scanning a focused ion beam over the surface to be analyzed. Each time it hits a pixel on the surface it causes “sputtering”, liberating molecules from the top surface of the material – in a cell, the outer cell membrane. The molecules enter the TOF mass spectrometer, giving us a mass spectrum for that one pixel. We repeat the process until we have generated a 2D image. We can then pull up the image for any of the mass peaks in the mass spectrum – for example, we might want to look at a particular lipid, drug molecule or metabolite. To generate a 3D image, we use another ion beam to carefully remove a thin layer of the surface, like a microtome slice. In a normal SIMS instrument, the material removed is discarded, but in OrbiSIMS it is analyzed by the Orbitrap, increasing our sensitivity and specificity. We then repeat the whole process for the newly revealed surface, before removing another layer.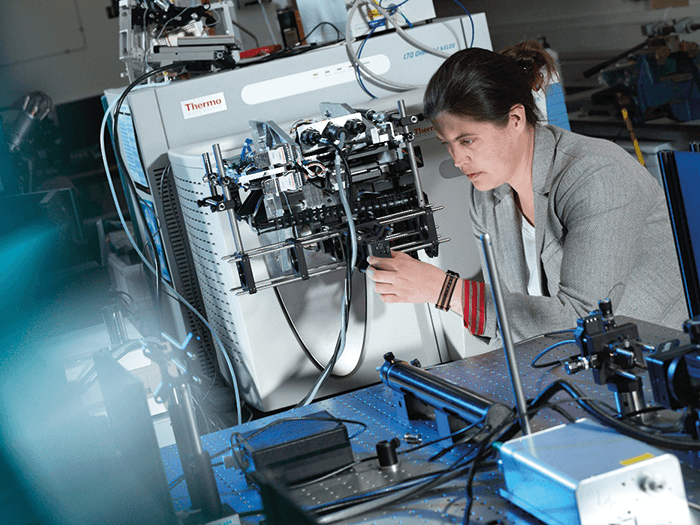
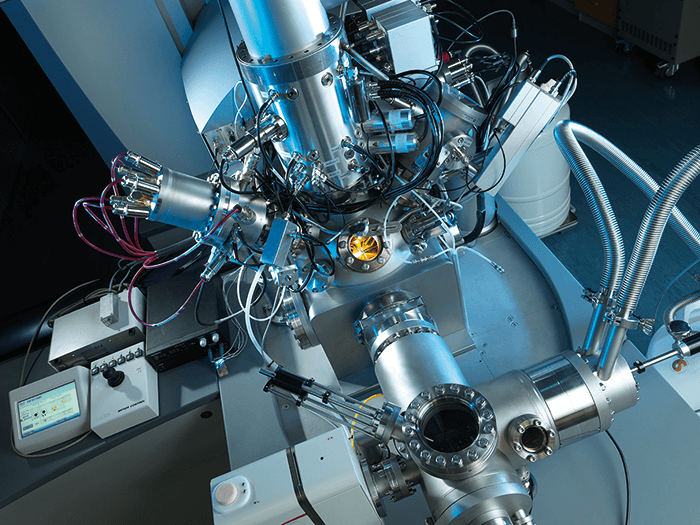
To put it another way – imagine you are digging in your garden. You take a digital photo of the plot you are about to dig, then get your spade and dig out a layer of soil, before taking another picture. If you keep taking photos as each layer is dug out, you eventually build up a basic 3D image of the plot. That’s SIMS. With OrbiSIMS, we not only take photos of the layers of soil as they are revealed (using TOF), but also analyze the soil that is removed (using Orbitrap technology). To make the instrument, we brought together two of the leading mass spectrometry companies – Orbitrap-maker Thermo Fisher Scientific and imaging TOF-SIMS specialists IONTOF. GlaxoSmithKline is an essential partner in the project – helping to ensure a successful outcome that will have impact in the pharmaceutical industry to reduce drug attrition and improve our understanding of drug up-take at a single-cell level.
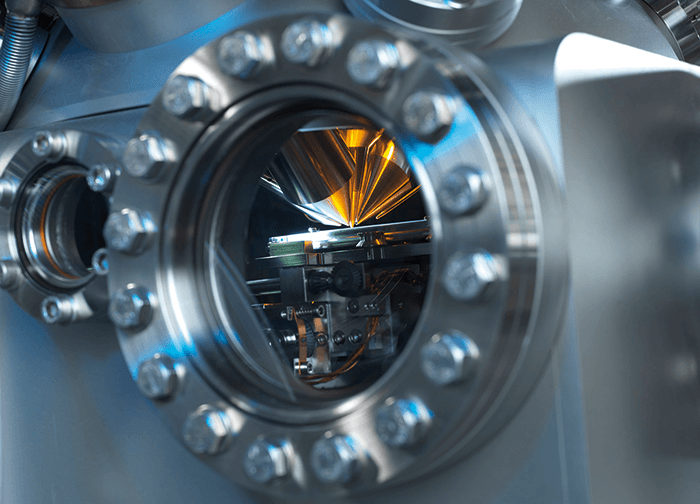
Sharing OrbiSIMS
The first results were presented in a plenary talk at the SIMS XX conference, Seattle, USA in September 2015. People were very excited when they saw what was possible with OrbiSIMS, and we are now working with a number of groups who plan to install an OrbiSIMS in their own labs. We have taken a great deal of care to make the workflow as simple as possible for people with a biology or life sciences background. Alexander Makarov made a huge contribution to the life sciences when he invented the Orbitrap, and it has become a de facto standard for proteomics and metabolomics studies. People are very comfortable with the technology and its capabilities, so the skills are already there to move into imaging with OrbiSIMS. If you can use an Orbitrap, you are halfway to being able to operate OrbiSIMS. To learn the imaging side of things takes longer, but people with some research experience can usually get up and running fairly quickly. Another nice feature of this instrument is what we call “cryo-SIMS”. If we want to examine cells down to the organelle scale, we need to be able to preserve the ultrastructure of the cells. Researchers in transmission and scanning electron microscopy have done so much beautiful work on preparing samples for a vacuum-based instrument – we have copied their achievements with pride. Our instrument is compatible with Leica sample preparation systems, so anyone with previous experience of TEM and SEM can easily prepare samplesNew insight
We took delivery of the first OrbiSIMS in November 2016. It has already started to give us some unique insights, and we have many more projects planned. The applications for OrbiSIMS are many and varied, but a big focus for us is the development of new drugs. There are three key questions we must answer about any potential drug:- Does the drug reach its target?
- Does it bind with the target?
- Does it have a pharmacological effect?