Peter: Hi Bob! The Analytical Scientist wants to get our thoughts on separation science - shall we talk now?
Bob: Let’s do it!
Peter: Sure. I guess I’ll start by saying that separation science constitutes arguable the most important group of techniques available to analytical chemists. These methods find a home in most application areas, but – as my job title indicates – I lend some focus to forensics. Separation techniques are uniquely important in this field… After all, DNA analysis also relies on these tools.
Bob: I couldn’t agree more. And, with the increasing volumes of data we are producing with such methods, my research focus – the interfacing of separation science with chemometrics – becomes ever more important. HPLC is a particularly robust and reliable technique across application areas, and our equipment demonstrates an incredibly low downtime.
Peter: Less than ten percent downtime, right?
Bob: Yes, and this compares favourably with our other equipment, such as our mass spectrometers. Overall, though, what I love about working in LC is the expertise and knowledge it requires. New challenges arise constantly, even for experts, and we are constantly provided with new puzzles to solve from industry.
Peter: Absolutely. And how do we keep our knowledge up to scratch? By visiting key conferences, like the annual HPLC meeting. I always take a number of students and postdocs to the event – it’s essential to get young scientists involved, and the culture for this group is particularly strong at EU meetings. And you also attend, Bob?
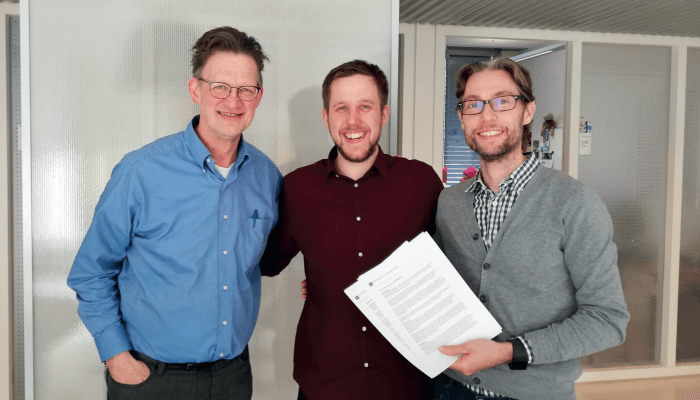
Bob: Of course! I always bring the research of both myself and the wider team. In lieu of the coronavirus situation, I’ve implemented a chromatography journal club between our lab and further groups, and this has been great – almost better than a real conference at times! But the great thing about HPLC is the shared interests and opportunities to exchange innovative ideas. We should prevent ourselves from working on islands – so to speak – wherever possible, and bringing the community together is the best way to avoid this. This is especially the case with the crowd at HPLC, which consists of both HPLC technology specialists and specialist users, who apply the technique to specific application areas.
Peter: And these application areas are so diverse, from pharmaceuticals (where HPLC has a major role in safe drug development), to medical diagnosis, food quality and safety, industrial materials (such as polymers), and so on. In fact, our own research in multidimensional separations actually feeds right into these polymer applications; comprehensive 2D-LC contributes significantly to this field, and is now an indispensable tool.
Bob: Right, and on this front, development of retention modelling of LC separations to rapidly compute optimal method parameters for 2D-LC is surely one of the greatest breakthroughs in the field thus far?
Peter: Absolutely!
Bob: Well, that and unique couplings of different instruments. For example, using reaction modulation for nanoparticle characterization.
Peter: And let’s not forget your own contributions to multidimensional separations, which now also encompass GC×GC.
Bob: Thanks, Peter – I’m flattered. Ultra-HPLC (UHPLC) has also been an indispensable tool in most of our 2D-LC research, both for high-resolution separations (dimension one) and for very fast separations (dimension two). Without UHPLC, 2D-LC would not be as powerful as it is today.
Peter: I couldn’t agree more… But let’s not forget where this all started some 50 years ago.
Bob: Hey I’ve got a question: how old would you say LC is in “human years?”
Peter: 40 years.
Bob: Really? I’d say 30 – it’s mature, but there’s still much more to come. And it all started with the pioneering work of Nobel Laureates Martin and Synge, who showed that HPLC required small particles to compensate for the low diffusion coefficients of liquids. This requires some pressure to give a reasonable flow rate and led to a great debate of high-pressure versus high-performance LC.
By Frantisek Svec, Department of Analytical Chemistry, Faculty of Pharmacy, Charles University, Hradec Králové, Czech Republic
My first encounter with chromatography dates back more than 30 years. My first projects focused on the application of polymers in separation science by developing a classic format of stationary phase – beads. But this format was soon replaced by a new approach: monoliths, of which we knew very little at the time.
The monolith boom came in the 1990s, and has continued growing since. My current position, supported by the STARSS (Specialized Team for Advanced Research in Separation Science) project, is exceptionally well placed for applying subtleties of separation science, and particularly chromatography.
This project means not only conducting excellent research using high-end instrumentation, but also teaching our students and postdocs. They are the next generation to whom we will hand over the baton, expecting them to love chromatography just as we do, and to discover creative solutions to society’s significant challenges, including environmental issues, food safety, affordable health care, and the development of drugs against new targets.
HPLC has developed significantly since its advent more than 50 years ago. Focusing just on the column technologies:
- 1966: Horvath and Lipsky used stationary phases with a particle size of around 50 μm packed in up to 275 cm long columns. The particle size has decreased ever since.
- 1990s: New columns format – monoliths – invented. Monoliths allowed HPLC separation at unprecedented speed – separating molecules as large as proteins in mere seconds.
- Today: Sub-2 μm particles are a current industry standard. Horvath’s idea of pellicular beads has transformed to give today’s core-shell particles. These developments have led to a significant increase in column efficiency, meaning excellent separations could be achieved with much shorter columns.
- Tomorrow: What can we expect to see in the future? Even smaller particles? This would require chromatographic hardware capable of tolerating higher pressures and dealing with associated challenges. Narrower columns? Perhaps, since narrower columns require smaller flow rates to achieve desired velocity, thus reducing consumption of the mobile phase and attempting “green” chromatography. Micromachined columns? Using processes typical of microelectronics, the preparation of columns containing arrays of pillars in a channel are emerging. 3D printing? I anticipate that columns will be produced this way. Early birds are already emerging on this front; printed monolithic columns will be designed in silico. However, high-resolution and rapid 3D printers are as of yet are unavailable.
Alongside column breakthroughs, detection methods have also taken impressive strides. The introduction of electrospray ionization to support MS by Fenn in the 1980s is a great example, and an essential breakthrough for the field of proteomics. Despite the availability of several approaches in the detection area, MS has become the most frequently used characterization technique for multistep analytical procedures. Significant improvements in MS instrumentation have been achieved in recent years, meaning spectrometers are both more accurate and more sensitive.
Yet, numerous sample preparation steps precede the chromatographic analysis. This area will remain a major target of studies for the foreseeable future, as the diversity of samples to be analyzed is close to infinite. Their preparation will require tailor-made, integrated, and high-throughput methods, alongside a decrease in both sample and instrument sizes, as well as automation, robotics, and multiplexing.
In short, there is much that must be achieved. Thus, the problems we face will not only keep us busy, but provide ongoing challenges for the next generations of analytical scientists and engineers.
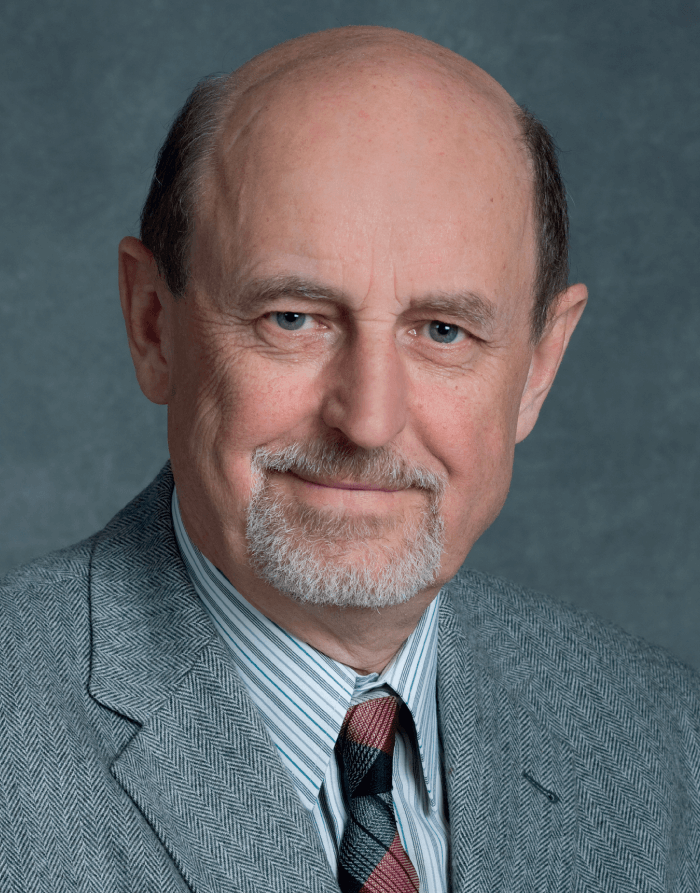
Peter: I was in high school back then, but a few years later began performing HPLC in the lab myself. We had a few Waters (M6000) pumps, and I probably didn’t appreciate how great they were. I guess I was spoiled from the beginning, and you’d need to ask people (even) older than me about the early ordeals. In any case though, the 1970s were a revolutionary time in LC – gradient elution was still a new concept, and so were chemically bonded phases. LC-MS was difficult, and we had multiple manual ways to integrate peaks, including a planimeter (a fabulous instrument), followed by cutting out and weighing the peaks after photocopying the recorder trace.
Bob: I read about those! All kinds of columns (stationary phases) and mobile-phase mixtures were tried with variable success. The development of chemically bonded phases was actually a major breakthrough. This culminated in a focus on non-polar octadecyl-silica (C18 or RP-18) phases and polar (water-based) eluents. This combination of polarities was opposite to the earliest HPLC studies (polar adsorbent and apolar mobile-phase), and thus became known as reversed-phase liquid chromatography (RPLC). The conventional normal-phase (or straight-phase) LC systems soon became an anomaly.
Peter: It’s no wonder – RPLC has many advantages. It offers immense flexibility (fully miscible solvents ranging from water to tetrahydrofuran), high selectivity and efficiency, rapid column equilibration, compatibility with aqueous samples (including biofluids) and MS, and many more. RPLC is there to stay; it is very unlikely that LC will ever return to normality.
Bob: Indeed, and decades of research have gone into positioning (RP)LC as the reliable and robust technique we know today. Even before your first dive into HPLC in the 70s, great scientists like Huber, Kirkland, Knox, Giddings and Horvath first laid the foundations for the technique.
Peter: And let’s not forget Guiochon and Snyder!
Bob: Of course! Today, alternate retention mechanisms steal only a small portion of the limelight. Hydrophilic-interaction liquid chromatography is fashionable, but it is useful only for very polar analytes. Ion-exchange chromatography remains important for ionic compounds, size-exclusion chromatography for polymers, hydrophobic-interaction chromatography for the separation of intact proteins, and supercritical-fluid chromatography has made a bit of a comeback, especially for the separation of chiral compounds. All these techniques have their niches, but RPLC occupies most of the playing field.
Peter: Very true – and likely because of the continued input given to improving the various aspects of HPLC technology. An impressive development on this front has been open-tubular LC (OTLC). Fundamentally, OTLC is attractive, provided efficient columns can be made with diameters of 10 mm or (preferably) less. Poppe’s group were among those that showed it was feasible, but the dynamic working range was grossly inadequate...
Bob: Which is why efforts in the field then mainly focused on effective, alternative packing materials, like monolithic columns and – eventually – pillar-array columns. Overall, packings have become much more efficient, reproducible and stable, and core-shell particles were developed to further enhance performance.
By Monika Dittmann
I worked at Agilent Technologies in Waldbronn, Germany, from August 1988 to November 2019 as a research scientist, specializing in HPLC, capillary electrophoresis and microfluidics. In this position I largely applied the fundamentals of separation science to the development of analytical instrumentation to serve as a consultant to the engineers (hardware, software and the like).
HPLC is the essential analytical technique used to develop and test drugs, test the safety of food, enable doping control, monitor the environment, and much more. Without HPLC, our modern life would be much less safe.
A number of breakthroughs have pushed the technique to this point: the application of high pressure (up to 400 bar) to LC some 40–50 years ago, which enabled the use of much smaller (5 µm) particles; development of reversed-phase stationary phases in the early 80s; the advent of ultra-high pressure instruments by Jorgenson in the late 90s; and the first commercial UHPLC instrument (Waters) in 2004 – others soon followed suit.
I am especially interested in modern UHPLC instruments – they are extremely versatile, and can be applied to almost any analytical problem. Over the past 15 years, we’ve witnessed vastly improved speed and resolution in these analyses, as well as those in LC×LC. Combined with MS detection, very challenging problems are now solved with relative ease, such as analysis of biological drugs. And, at present, it’s believed that further increases in operational pressure or reduced particle size will have only marginal benefits.
Today, LC instruments are becoming increasingly usable by non-experts, and may eventually become a common commodity, like a TV or a car. This, of course, requires easy-to-use, smart instruments, improved diagnostic features and support in application areas. In line with my work, prediction and simulation of separations could lend support to application development and user training, but large databases of retention data are required – this is maybe best collected through an open-source project.
Open-source projects could also support us in overcoming current bottlenecks in research – particularly, method development and data analysis – that can be solved computationally and require close collaboration between analysts and computer scientists. This is already happening in some academic groups and companies. The coronavirus pandemic is clearly a testing time, but perhaps it has something to teach us about the true value of collaboration and data sharing to speed up progress – in LC and elsewhere.
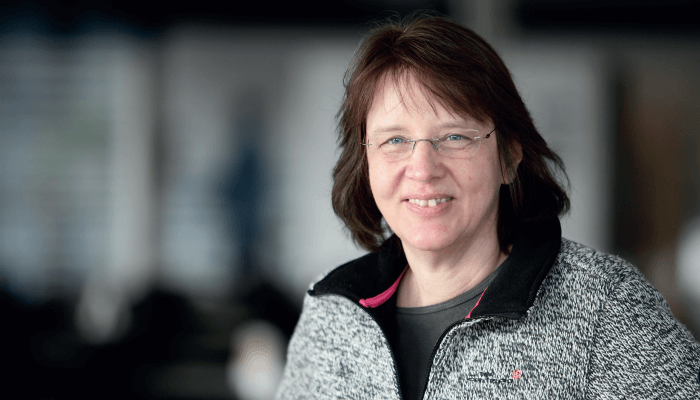
Peter: On the topic of enhancing performance, the advent of UHPLC spurred a major jump in technology and applications. With higher pressures, UHPLC allowed smaller (sub-2 μm) particles to be used for very fast analysis, and also forced overall improvements in instrumentation. Like you said before, Bob, the technique is now...
Bob: Indispensable!
Peter: Yes, indispensable – and found in virtually every analytical lab today.
Bob: And what about the future?
Peter: First, we need to open the door to LC for non-specialists.
Bob: You said it. But this is – obviously – a major challenge for instrument and software manufacturers alike. We need intelligent software to combat the fact that the number of LC instruments is growing much faster than the number of trained specialists.
Peter: Plus, the expertise required is becoming increasingly complex. On one hand we require more expertise, but we cannot train experts at the rate at which they are needed. This dilemma we have to address with very smart artificial intelligence.
Bob: Smaller systems are also a priority. The volumes of organic solvents we use right now are too large, so that movement towards miniaturization is inevitable. Simpler, automated systems are also desirable, as you say, but in this case, we must sacrifice some efficiency for a selectivity benefit. Separation science must also move out of the lab to help protect against environmental issues and improve society.
Peter: I agree – just look at the coronavirus pandemic, for example. Separation science will surely play a pivotal role in finding a solution, just as it does in the monitoring of environmental pollutants.
Bob: Well, it’s been great chatting, Peter. But it looks like it’s time to get back to the lab!
Peter: Is that the time already? We should do this again sometime.
Bob: Absolutely.