Breath Analysis for Disease Diagnosis
Jumping on the breath biomarker bandwagon is tempting, but a number of failures to observe the fundamental rules of good science have cast doubt over the technique. Here, the authors describe best practice, from collection to clinical evaluation.
Terence Risby, Lisa Spacek |
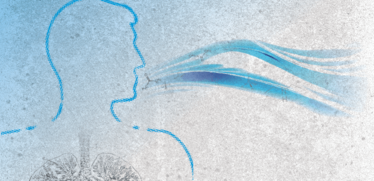
The current resurgence of interest in breath analysis follows directly from Nobel Prize winning discoveries of “nitric oxide as a signaling molecule in the cardiovascular system” and identification of significant concentrations of nitric oxide in human breath. Because the analysis of exhaled breath is relatively easy to perform, it’s ever more important to encourage high quality investigation. The use of breath analysis in clinical diagnosis requires careful attention to be paid to:
- Breath sampling
- Breath analysis
- Biomarker discovery
- Clinical evaluation of potential breath biomarkers.
Failure to consider each of these important factors will limit their subsequent utility and increase the likelihood of “garbage in and garbage out”.
Researchers who proposed that breath nitric oxide could be used to evaluate airway reactivity in patients with asthma have paid careful attention to each of these factors and, as a result, the US Food and Drug Administration (FDA) has approved breath nitric oxide as a diagnostic tool to monitor asthma therapy.
Breath Sampling
Collection of a representative alveolar breath sample under controlled conditions is an a priori requirement for successful breath analysis research. Ideally, breath should be sampled when the concentration of carbon dioxide reaches a plateau and it is important that the patient does not hyperventilate. Normally, the depth and frequency of breathing are under autonomic control. However, when asked to provide a breath sample, the patient often transitions from autonomic breathing to conscious breathing and consequently hyperventilates. The easiest way to minimize hyperventilation is to ask the patient to breathe at a defined frequency (for example, 10 times per minute) and a defined tidal volume using visual and audio prompting. This protocol for breathing is known as paced breathing.
Sampling multiple breaths improves the accuracy of the resulting analyses and breath samples can be collected in inert gas-sampling bags, made from Tedlar, Teflon, Mylar, etc. The requirements for these gas-sampling bags are that they must not adsorb breath molecules and have volumes that exceed the amount of breath to be collected by at least a factor of two. This volume restriction minimizes backpressure from the collected gas that will variably restrict exhalation. Similarly, the inlet to the sampling bag should have sufficient dimensions to minimize pressure drop across the inlet. Evacuated polished stainless steel cylinders or thermal desorption tubes packed with adsorbents or solid phase microextraction (SPME) tubes, are alternative media used for the collection of breath samples. Sampling with these devices is performed indirectly using constant flow or constant timed gaseous diffusion. The advantages of these latter devices are that the study subject experiences no resistance to breathing. Moreover, the breath samples are easily transported in these devices from the sampling site to the laboratory.
Breath Analysis
After collection, samples of breath can be analyzed with any analytical chemistry technique with specialized inlets. Many published studies have used standard analytical chemistry methods, such as capillary gas chromatography (GC) with sensitive, selective detection systems based upon optical spectroscopy or mass spectroscopy, since capillary GC can separate complex breath profiles and make biomarker discovery easier. Two-dimensional capillary GC improves the separation of the complex breath matrix further.
Many of the more modern analytical methods based upon absorption spectroscopy, selective ionization mass spectroscopy with ion-molecule reactions, electro-chemistry, and chromatography, now have sufficient speed, sensitivity, and selectivity to allow real-time breath analyses to be performed – and analysis can be based on a single breath. Therefore, sampling protocols have been developed that have the patient breathe at a constant flow rate of exhalation by imagining they are trying to whistle a musical note. Flow can be monitored indirectly by monitoring mouth pressure. Visual prompting of mouth pressure allows the subject to breath repeatedly at the same flow rate.
Biomarker discovery
The molecular profile of any breath sample will be defined by the concentrations and identities of the volatiles in inhaled air and in blood. The volatiles in air can be derived from both ambient and indoor sources. The sources of the volatiles in blood are:
- Molecules and/or their metabolites that have been inhaled
- Molecules and/or their metabolites that have entered the bloodstream via the skin
- Molecules and/or their metabolites that have entered the bloodstream from ingested foods or beverages
- Molecules produced by foreign cells, such as viruses, bacteria, fungi, and yeasts
- Molecules produced by tissues in the body including the mouth, nose, sinuses, airway, and gastrointestinal tract.
The majority of alveolar breath (99.995 percent) consists of nitrogen (78 percent), oxygen (13 percent), carbon dioxide (5 percent), water vapor (4 percent); the inert gases and the remainder (<50ppm) is a mixture of as many as 1000 different compounds. The rates of excretion of molecules in breath are directly related to rates of ventilation and cardiac output. The physical and chemical properties of molecules also affect their rates of excretion. If a molecule is lipid-soluble, it may be stored in tissues not well perfused by blood, such as adipose tissue, and thereby be released more slowly than a similar molecule with hydrophilic properties that is not stored.
In general, the concentrations of endogenously-produced molecules are lower in breath than the concentrations of molecules from exogenous sources. Unique molecules in breath can only originate from the ingestion, inhalation, or dermal absorption of exogenous substances or be metabolically produced by foreign cells (bacteria, viruses, or eukaryotic organisms). Normal cellular biochemistry can only be induced or suppressed by abnormal physiology and although disease states may appear to be producing unique molecules, these results may only be a reflection of the detection limit of the analytical method.
Clinical evaluation
The use of breath for disease diagnosis first requires that the concentration profiles of breath molecules for normal healthy human subjects be established and these studies must include such variables as age, gender, ethnicity and body mass index. A methodological approach entails univariate analyses where each one of these characteristics is compared to breath biomarker values. This process will define breath measurement confounders, or participant characteristics that change measured breath values. It may be that an adjustment accounting for one or more of these characteristics is necessary before comparisons can be made. For instance, standardization of any biomarker molecule that is measured in exhaled breath can be corrected by average concentration of mixed expired CO2. This serves to adjust for anatomic, physiologic, and instrumental dead space based on each individual participant. If comparisons of the results of breath analysis are to be used to study therapeutic intervention or to compare different study subjects, it is important to standardize concentrations of breath molecules to oxygen consumption or carbon dioxide production.
To recognize potential confounders, well-described cohorts of participants identified as “healthy” or “diseased” will need to provide breath samples that are evaluated for validity and reproducibility. Large study groups are required so that unknown confounders can be detected and explored. Participants should be identified who exhibit either significantly high or low breath biomarker concentrations that increased variability in a presumed homogenous population. Further research is needed to clarify our understanding of attributes that contribute to breath biomarker variability. Potential confounders, such as dietary intake immediately and within eight hours of breath collection, and mouth rinses done immediately prior to breath collection, must be evaluated. Collection and analysis of such data will refine the application of breath biomarker measurement in diverse populations.
The use of a particular breath molecule or group of molecules to ascertain disease can be implemented once a threshold, which separates healthy individuals from those with disease, is identified. The breath molecule will need to be compared to the current reference or ‘gold standard’. Notably, a true gold standard test may not be available. The classification of disease versus healthy in dichotomous outcomes or multiple levels of disease severity will require sample sizes large enough to ensure adequate power and precision. Complete and accurate reporting of the accuracy of diagnostic tests is critical and guided by the Standards for Reporting of Diagnostic Accuracy (STARD) initiative first promulgated in 2003.
Despite its promise and the fact that there have been a large number of publications on breath analysis, only a handful of tests are used clinically, with a few others used for investigational purposes. Approaches to clinical breath analysis fall into two basic categories: tests based upon the quantification of metabolites in breath after administration of a labeled drug or substrate; and tests that quantify molecules in breath without any prior administration of a drug or substrate.
Labeled breath tests
Carbon dioxide, one of the major products of cellular respiration, is the most popular metabolite investigated. Briefly, substrates labeled with carbon-13 (13C) are metabolized to 13C-labeled carbon dioxide that can be separated spectroscopically from endogenously produced carbon dioxide by non-dispersive infrared absorption spectroscopy or isotope ratio mass spectrometry. The analytical limitation of this approach is the natural abundance (1.1 percent) of 13C-labeled carbon dioxide. Breath tests based upon this approach require that the rate of metabolism of the 13C-labeled substrate and the rate of excretion of carbon dioxide be well characterized. Typically, a set dose of a labeled substrate is given to the patient to evaluate a disease-related metabolic change. The concentration of 13C-labeled carbon dioxide is quantified after a defined time. The labeled substrate is selected for a specific biochemical pathway that is related to a specific disease.
It is also possible to identify genetic polymorphisms of patients on the basis of the absence of a specific enzyme by using the required labeled substrate for this enzyme. The limitation of tests based upon this approach is the ability to identify a relevant biochemical pathway that will provide disease-specific diagnostic information and the design and synthesis of a suitable labeled substrate.
Irrespective of the biochemical pathway under investigation, the breath test must be performed under carefully controlled conditions after a fixed fasting time. Time between the administration of the labeled substrate and the administration of the breath test must be defined. Currently, the urea breath test for Helicobacter pylori, an etiology of chronic gastritis and gastric ulcers, is the only FDA-approved labeled breath test.
Endogenous breath tests
The most widely used clinical breath test is based upon monitoring the concentration of unlabeled carbon dioxide, a major product of respiration. This test, know as capnography, is the instantaneous measurement of the concentration of carbon dioxide as a function of time. The capnograph provides real-time information for the excretion of carbon dioxide. The shape of the curve provides information on alveolar ventilation and respiratory patterns. Lung diseases, such as chronic obstructive pulmonary disease (COPD) and asthma, affect these breathing parameters. Capnography is used to confirm the correct placement of endotracheal tubes (tracheal intubation) in all operating rooms, intensive care units and any situation that requires assisted ventilation. There are a number of companies that manufacture capnographs and most are based upon of the absorption of non-dispersive infrared radiation. In certain clinical situations, use of these portable and inexpensive devices has become standard of care and neglecting to use capnography can be construed as medical malpractice.
Assays based upon the determination of breath hydrogen or breath methane are the next most popular clinical tests. These tests are conceptually similar to the labeled breath test. Briefly, the concentration of hydrogen or methane is determined in the breath of fasting patients at defined times after the administration of a low dose of a specific sugar (fructose, lactose, glucose, dextrose, sucrose, d-xylose, sorbitol or lactulose). Elevated levels of hydrogen or methane after administration are indicative of irritable bowel syndrome and various sugar intolerances. Hydrogen and methane were initially determined using a specifically designed gas chromatograph but, more recently, hydrogen is monitored with membrane electrodes, which are hand-held and significantly less expensive.
The final breath test is based upon the determination of nitric oxide. The physiological origins of this reactive species are inflammatory cells in the oropharyngeal cavity and cells within the respiratory tract. Currently, breath nitric oxide is used clinically to monitor therapy for asthma. Initially, nitric oxide was determined by monitoring the chemiluminescent reaction with ozone. More recently, commercial breath nitric oxide monitors are based upon the absorption of mid-infrared radiation or use membrane electrodes. The latter monitors can be hand-held and less expensive.
In conclusion, clinical breath analysis remains in its infancy, despite the fact that its potential has been recognized since antiquity. Recent advances in instrumentation are inspiring a renaissance in breath analysis; in particular, the wider availability of real-time, portable monitors is enabling breakthrough research that can more easily allow the analysis of large numbers of human subjects.
Efforts to translate technical knowledge into clinical applications require expertise in several areas. Only dynamic multidisciplinary teams, consisting of device makers, breath analysis experts, and clinical researchers and clinicians (including statistical support), that bring together the specialized skill sets and problem-solving capability necessary can anticipate breakthrough success. Teams that lack any of these essential roles will not be successful.
Future research efforts must better define the pathophysiologic mechanisms to which breath analysis can be applied, such as the contribution of gut flora to health and disease. Additionally, clinical studies are needed to define disease states that clinical breath analysis can diagnose. The unique contribution that breath analysis brings to patient care and clinical diagnosis merits ongoing work to establish original applications of this innovative technology.
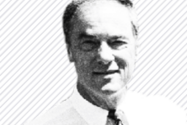
Terence Risby is professor emeritus in the Department of Environmental Health at the Johns Hopkins University Bloomberg School of Public Health.Terence received a PhD in Chemistry from Imperial College of Science Technology and Medicine London in 1970. His post-doctoral fellowships took him to the University of Madrid and the University of North Carolina. Terence’s current research interest is the use of breath biomarkers in clinical molecular epidemiological studies.
Lisa Spacek is Adjunct Assistant Professor at Johns Hopkins School of Medicine. During her fellowship and assistant professorship in Infectious Diseases, Lisa conducted clinical epidemiologic research focused on treatment of HIV infection in resource-limited settings including Kampala and Rakai in Uganda. Her expertise is in the development of clinical diagnostic algorithms and evaluation of clinical diagnostic tests. Lisa is a Diplomate in Internal Medicine and Infectious Diseases of the American Board of Internal Medicine, and has published 21 articles in peer-reviewed journals.