Catalyzing Innovation
The story behind the Polyarc® system – and how it sparked a discovery to make car tires from sugar.
Charlotte Barker, Paul Dauenhauer |
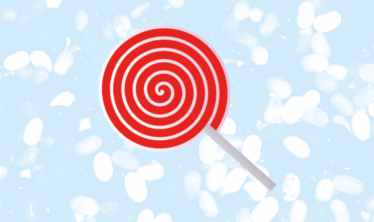
The Problem
Our group aims to produce renewable chemicals from biomass, which involves analyzing complex organic molecules with a lot of heteroatoms (atoms that aren’t carbon or hydrogen). Heteroatoms change the response rate, and so GC-FID of these molecules necessitates careful calibration. For each compound, you have to buy a standard (if one is available – many aren’t) to define the response rate – or use a crude estimate. We needed to find a better way.
Background
The first chemical engineering lecture I ever attended was on the Haber process, which combines nitrogen from the air with hydrogen to produce ammonia. Before its discovery in 1904, the world was heading for mass starvation because agriculture was failing to keep up with population growth. Easy access to ammonia-based fertilizers gave us the means to grow enough food for everyone, but it has also had serious environmental consequences. Nevertheless, it’s a chemical technology that has truly changed the world. I was inspired.
I wanted to work in a field with the same potential to change the world, and focused my attention on renewable chemicals. There is enough biomass available, the economics make increasing sense as glucose prices fall, and I saw a chance to get ahead of the wave.
My group is made up of engineers, but we’re also analytical chemists by necessity; the analytical challenges in producing renewables are immense. One of those problems is the difficulty of analyzing heteroatoms by GC-FID. Because our sample mixtures contain a large number of different heteroatoms, you can end up with a chromatogram with anything from 20 to 200 peaks. The time and expense of all that calibration meant that it just wasn’t feasible to study the hundreds of compounds we were interested in. Instead, we were forced to work with very simple systems followed by extrapolation. But given that the response factor of these heterogeneous molecules can vary from 0.01 to 1.2, estimates are not very effective!
The problem with FID when it comes to quantification is that its response factor is dependent on molecular structure. I was chatting to one of my graduate students about the problem one day, when we hit upon a solution. It occurred to us that we could identify and quantify the compounds separately. For quantification, we only needed to figure out the amount of carbon, while ignoring the structural information – and we could use an independent method, such as mass spectrometry, for identification. We figured that if we converted everything to methane before the FID, we would eliminate the impact of molecular structure on quantification and end up with a linear response. Although it was a very simple idea, putting it into practice was a real engineering challenge.
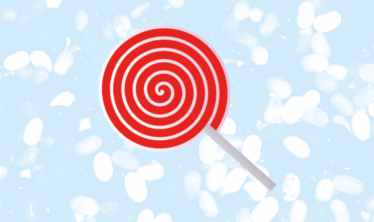
Sugar Rush
For us, the technology behind Polyarc has made a huge difference to our work. In fact, it helped us on the way to a major breakthrough – a process for manufacturing isoprene (the principal component of car tires) from glucose, rather than from petroleum.
It’s a three-step process:
- Glucose > Itaconic acid
- Itaconic acid > 3-methyl-tetrahydrofuran
- 3-methyl-tetrahydrofuran > Isoprene
The first two steps are simple enough – itaconic acid is already commercially available from glucose fermentation, and sequential hydrogenation produces 3-methyl-tetrahydrofuran (3-MTHF).
The final part (dehydra-decyclization of 3-MTHF) was the real challenge. First, we assessed all the conventional catalysts – none could get over 40 percent selectivity. The breakthrough came when we identified a new catalyst with a 90 percent selectivity. The resulting isoprene is identical to that found in synthetic rubber tires.
The Polyarc system greatly enhanced this research in two ways. First, our initial experiments produced many side products, each of which was readily quantified with the Polyarc system. Second, our experiments were conducted using a high-throughput experimental system that could measure up to 100 data points per day. The Polyarc system enabled rapid screening and analysis of a large number of complex mixtures to help us determine the conditions which gave 90 percent yield.
The ability to produce isoprene from a renewable source comes with many benefits. We know that there is a finite amount of petroleum, and as supplies run short, costs will rise. By using grass, corn or wood to produce isoprene, we essentially take CO2 out of the air and into the product – a form of carbon sequestering. Domestic production is another big advantage. I’m from Minnesota, where the two big industries are corn and forestry. Both industries are under pressure, and keen to find new and profitable uses for their crops.
Our detailed techno-economic analyses reveal that the most important cost driver is selectivity, so we are putting the majority of our efforts into pushing selectivity as high as we can. The process is now up to 90 percent selectivity and we continue to work on that, while we talk with several companies about commercializing the technology. Moving up to an industrial scale will be a challenge, but we’re engineers, so that’s our bread and butter.
The Solution
We start with a mixture of complex molecules, and pass them through a GC column. After the separation has occurred, instead of the column feeding into the FID, each molecule goes into a microreactor that converts every carbon-containing molecule to methane via dual reactions of combustion followed by reduction to methane. The second half of this process (CO2 to methane) is similar to commercial methanizers; however, the initial combustion step means you can convert all organic compounds to methane, whereas a methanizer can only convert CO and CO2. The system can be used to replace existing methanizers, but it has significantly more capabilities. The reaction can be represented as:
Organic compounds + Air + H2 → Methane (CH4) + Non-carbonaceous byproducts
The methane goes into a conventional FID detector, where the response is proportional to the amount of carbon. Now that all molecules have been converted to methane the response rate is the same across the board, so there is no time-consuming calibration. Non-carbonaceous byproducts elute as volatile compounds. For example, sulfur will first be oxidized to sulfur dioxides and then reduced to hydrogen disulfide. For this reason, the Polyarc catalyst cartridge is designed with a long lifetime, to account for the impact of poisoning over time.
Conceptually, it’s very simple – but there were two major challenges in producing a useable system. First, we had to identify the thermodynamic conditions that would ensure a greater than 99.9 percent conversion to methane. This was addressed using a global thermodynamic calculation of all possible molecular structures. Second, we needed a sufficient catalytic conversion to approach equilibrium conversion to methane. To achieve this we combined catalyst and reactor design for maximum catalytic activity. We created a prototype – an ugly, Frankenstein’s monster of a machine made up of a steel block with straight holes connected with tubing and fittings. Despite problems with mixing and long residence time, the prototype proved very useful in our work.
The Polyarc: Applied
The Polyarc system has now found applications well beyond its original use for analysis of renewables. For example:
- Flavor and fragrance analysis of compounds where commercial standards are unavailable or prohibitively expensive.
- Extractables and leachables analysis using mass spectrometry and a Polyarc/FID to expedite analysis while increasing accuracy.
- Forensic analysis of synthetic drugs where the addition of Polyarc has enabled rapid quantitation in addition to the existing qualitative analysis.
- Bulk and specialty chemical analysis of complex mixtures to reduce calibration procedures, due to many of the compounds of interest having boiling points below room temperature.
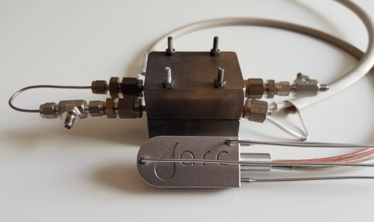
The original prototype (top) that eventually became the Polyarc (bottom).
I have known Activated Research Company (ARC) CEO Andrew Jones for around 10 years and when he visited my lab in 2014 our “monster” caught his eye. After I’d explained how it worked, Andrew pointed out that others might find our approach useful. We naively thought it was just a relatively simple solution to our specific problem and so it hadn’t occurred to us that there might be a commercial success story waiting to unfold.
To cut a long story short, ARC turned our prototype into a slick device – the Polyarc® system. The principle is the same, but ARC introduced many improvements; in particular, using 3D metal printing to produce the microreactor – a world first. ARC also focused on minimizing dead-volume in the microreactor to ensure the chromatography is maintained as much as possible, and designed a system that can be easily integrated into any GC set-up. The elimination of dead volume and mixing with the 3D printed metal microreactor helps to maintain chromatographic resolution, retaining nice, sharp peaks that are easy to integrate and quantify.
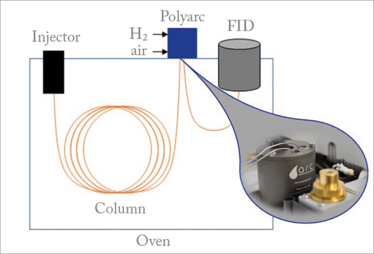
Figure 1. How the Polyarc system works.
Beyond the Solution
The Polyarc system has been commercially available for nearly two years now, and I regularly get calls from people asking for advice on applications or operation. People are finding new applications that I had never thought of (see box: The Polyarc: Applied). I initially conceived of the technology as a means to analyze compounds with no reference standard, but there are other labs who would prefer not to store standards; for example, forensic labs analyzing illegal drugs.
Award-Winning Innovation
The Polyarc system was recognized in the 2015 Analytical Scientist Innovation Awards (TASIAs), with the judges commenting: “it solves a problem as old as GC.”
The Innovation Awards were set up to celebrate innovation from companies big and small, during the preceding 12 months. Have you got a breakthrough you’d like to shout about? Contact the Editor ([email protected]) to find out how you can enter this year’s TASIAs.

After studying biology at Imperial College London, I got my start in biomedical publishing as a commissioning editor for healthcare journals, and I’ve spent my career covering everything from early-stage research to clinical medicine.