CD-MS: To Megadalton and Beyond
Charge-detection mass spectrometry (CD-MS) has extended the range of MS to gigadalton-sized viruses and polymers; and with a commercial instrument in development and exciting new applications in complex protein mixtures, maturity beckons
Martin Jarrold | | 10 min read | Technology
The origins of CD-MS date back to the 1960s. During the Sputnik era, as satellites were being launched into space, there was concern about the impact of dust on these satellites. Researchers aimed to accelerate particles to high velocities and then impact them onto surfaces to observe the effects. As a result, the concept of measuring the m/z and charge of a particle by passing it through a conducting cylinder was introduced. Then, in the mid-1990s, Henry Benner and colleagues adapted this idea by adding an electrospray source to study electrosprayed ions. Their approach involved single-pass measurements, which limited the size of the particles they could analyze to very highly charged particles in the megadalton range.
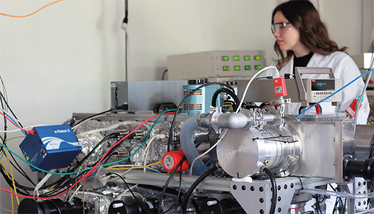
But my CD-MS story starts in 2006 – basically because I was looking around for something different to do. I had colleagues here in the chemistry and biochemistry departments at IU who were looking at viruses and I thought I could try to use mass spectrometry to measure their masses.
Of course, with conventional mass spectrometry, there’s a mass limit to what you can measure – usually around a megadalton, if you’re lucky, and if you have a fairly homogenous sample. But many samples are either heterogeneous or have high molecular weights, such as vaccines or viruses.
As is often the case when you start something new, we had many failures to start with. Then I came across several publications by Benner, who was then at the Lawrence Livermore Lab, on charge detection, which seemed perfectly suited to the task at hand.
To understand how charge detection works, we have to go back to the limitations of conventional MS, where you’re measuring the m/z spectrum. If you have a large, multiple charged ions, you also have to figure out what the charge is. You can do that if you have well resolved peaks in the m/z spectrum, thanks to the ions being in different charge states. But if you have a heterogeneous sample, each mass in the sample gives you a distribution of charge states, which overlap – and you end up with a featureless blob. You can’t figure out what the masses are because you can’t figure out what the charges are. With CD-MS, you perform a single-particle measurement, which gives you the m/z and charge for each individual ion. The m/z multiplied by charge gives you mass, so you have the mass of each individual ion. Once you do that for thousands of ions, you can take the masses and bin them into a histogram – that’s your mass distribution.
In other words, by individually measuring each ion’s charge and m/z ratio, you avoid the confusion caused by overlapping signals, allowing for the measurement of large particles and heterogeneous samples. The problems with CD-MS at that time was that it was limited to highly charged ions, and it had very poor resolution – in a nutshell, it wasn’t practical. I thought, we should try it, because it seemed to be such a cool technology, and I was sure we could find a way to make it better. So, we started doing CD-MS with a simple, single detector setup – with the ions just flying through. As soon as we had a working instrument a retired colleague, George Ewing, heard about what we were doing and got us interested in looking at the charge on water droplets. It was a good match because the CD-MS detectors we had in those days were really poor and highly charged water droplets were one of the few things we could measure. George led us astray and kept us distracted with water droplets for several years.
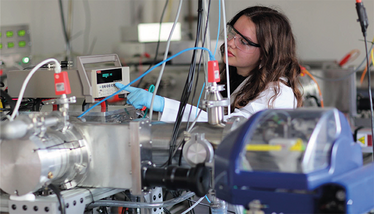
“You really need to start a company”
It took us close to a decade to really build upon Benner’s work and engineer the CD-MS technology into a practical measurement tool – it wasn’t until 2013 that we got our first ion trap CD-MS to work. The key improvement was the ability to measure the charge accurately, which had hindered Benner’s measurements. An important development here was using Fourier transforms to analyze the data. Initially, we used Gaussian signal processing, but incorporating Fourier transforms significantly expanded what we could analyze. Suddenly, we could examine much more lowly charged particles, and the frequency measurements obtained were a much more reliable and accurate way to determine the m/z. From that point on, our focus shifted to increasing the precision of charge measurement and ensuring the accuracy of frequency measurement – an engineering challenge that involved extensive simulations to optimize the process. We became proficient at conducting computer simulations, diligently working to achieve a level of accuracy and reliability sufficient for commercial use.
As we were working on this, David Clemmer – who I’ve known for many years and who now works with us at Megadalton – was always telling me that we really need to start a company and commercialize our CD-MS technology. I would always reply: “Yeah, right! I’m a chemistry professor, not a businessman!” But around 2018, we began getting interest from people in the pharma industry using AAV vectors for gene therapy.
When researchers are encapsulating DNA inside a viral vector, one of the first analytical questions is the ratio of empty to full particles and how much partial packaging is going on. Given the size of the particles, answering this question is quite challenging. You might have a selection of capsids ranging in mass from 3.5 to 5 megadaltons (for example), depending on how fully packaged they are. With CD-MS, you can accurately determine the ratio of full-to-empty capsids in some detail. In fact, you can glean quite detailed insights into the protein composition of the AAV capsid, as well as the DNA inside the capsid, too. But more on that later.
Initially, we were fulfilling requests to measure samples for free – just as something on the side. But I realized this wasn’t the best use of student’s time, so we started charging people for it and paying students on the side to do the measurements. The requests kept coming and we just couldn’t keep up. David’s comments about commercialization started to make a great deal more sense!
We started the company, Megadalton, in 2018. Ben Draper, a graduate student of mine, was the first employee. In fact, he was all set to work for Intel, until we offered him less money to stay… Thankfully, he accepted! He’d been working on the technology and was excited to get involved in trying to commercialize it, and he has done a fantastic job developing the analytical capabilities of CD-MS.
Waters supported us from the get go – initially giving modest funds to keep us afloat, before we were measuring enough samples to survive on our own. They continued to invest and eventually visited to check out our instrument, had their own scientists run it, and so on. In the end, they acquired the IP from Indiana University and Megadalton in 2021, and we’ve continued to work closely with them.
They’ve been building prototype instruments – what they call “technology demonstrators.” And they’re currently working on a version of the instrument that is close to the one they’ll end up selling. The projected date for the instrument to hit the market is, I believe, sometime in 2025. So watch this space!
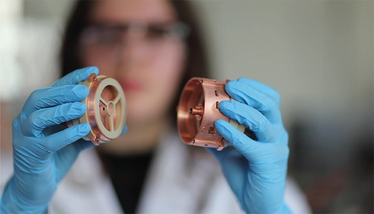
Maturity beckons
CD-MS has come a long way, but it is by no means a mature technology. I think we’re going to see the resolution of the m/z measurement get much better – this is what we’re focused on. And when you’ve got a technique where you can measure m/z very accurately, and you can assign a charge state to that m/z, you can look at very complex protein mixtures. This wasn’t one of the original ideas behind CD-MS – it was really to look at big things. But I think it’s going to look at medium-sized things as well – and have a profound impact there. For example, I think it will soon become possible to take a sample of serum and, with very little cleanup, electrospray it, and then look at it with CD-MS to get a readout of what proteins are there.
Going back to the original intention, CD-MS has extended the range of mass spectrometry from around a megadalton, depending on the sample, to around a gigadalton. Now we can look at very large particles, such as polymers and very large viruses.
I mentioned the demand for a technique like CD-MS in AAV analysis, but AAVs are actually relatively small compared with other viral vectors, and they are also limited in terms of the size of the genome that they can encapsulate. So, there’s interest in using larger viruses to act as gene therapy vectors, such as adenoviruses and lentiviruses. CD-MS can analyze these much larger viruses; we’ve published an analysis of a 150 megadalton virus, for example.
We’ve also done some CD-MS work on the COVID-19 spike protein, which is another example of its utility. The spike protein is very heterogeneous due to the 22 glycosylation sites on each of the three trimers, resulting in a total of 66 sites. This creates a heterogeneous mixture of masses that conventional MS cannot analyze. However, CD-MS can measure the mass distribution, even though it’s broad and cannot yet resolve individual components with very high resolution.
We have also started investigating lipid nanoparticles, spurred by the success of COVID-19 vaccines, which led to increased interest in their other applications. Using CD-MS, we have also measured the masses of DNA, particularly looking for genome truncations in AAVs. By extracting DNA from gene therapy vectors and measuring its mass, we can determine if it matches the expected mass. If the DNA is shorter, it indicates truncation, which is another significant issue in AAV gene therapies.
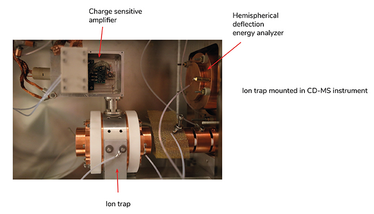
Recently, we began examining antibody binding to AAV. Conventional methods cannot resolve individual mAbs binding events due to the heterogeneity of AAVs. However, CD-MS can measure the mass distribution, providing the average number of mAbs binding and an estimate of the binding distribution. This distribution can offer insights into the binding mechanism – information not accessible through other techniques typically used to investigate ligand binding.
It’s an exciting time for CD-MS. We’ve recently significantly reduced the time it takes to measure the charge accurately. We’re now at a threshold where we can truly improve the m/z resolution, which will, in turn, enhance the mass resolution. For a long time, we were stuck at a resolving power of around 700. However, we’ve recently improved the resolving power to 14,000. I believe we can push this up by another order of magnitude, making CD-MS competitive with very high-resolution MS technologies. I’m very excited about the next couple of years. I think these advances are achievable within this timeframe.
There are still issues to resolve, such as adduct formation with samples as we push mass resolution to these high values. If ions have extraneous substances attached, it will lead to broadened mass peaks and inaccurate measurements. Despite these challenges, I believe they are solvable, and we have the prospect of achieving very high mass resolution on large complexes.
Another important advance has been the recent development of high throughput CD-MS where spectra can now be measured in less than a minute. This has allowed direct coupling of LC to CD-MS. The coupling of ion mobility and CD-MS is also just around the corner.
Is there an upper limit to the size of particles we might be able to analyze using CD-MS? At some point, as particles become larger, gravity will start to play a role. We’re not at that stage yet, and it’s not clear if measuring into the gigadalton range, such as measuring cells, is necessary. Personally, I’m not yet convinced that exploring tens or hundreds of gigadaltons is essential. Having said that, a few decades ago, people likely had similar doubts about the scales we currently work with, so perspectives could well change in the future.
Images Credit: Supplied by Author
Distinguished Professor and Robert & Marjorie Mann Chair, Indiana University Bloomington, USA