Digging into Biomarkers of Oxidative Stress
Hyphenated chromatography techniques enable the selection of representative biomarkers of oxidative stress – and help resolve the huge complexity of lipid oxidation products.
Our bodies’ cells are under constant threat from various toxic agents, including reactive oxygen species (ROS) formed by normal physiological processes. Fortunately, our antioxidant defense systems can inactivate them. However, under certain conditions, prooxidative/antioxidative homeostasis may get out of balance and excess ROS may be present in cells – a condition known as oxidative stress.
Oxidative stress is a prominent feature or a secondary effect in many acute and chronic diseases, the list of which is almost endless: atherosclerosis, asthma, chronic obstructive pulmonary disease (COPD), Alzheimer’s, amyotrophic lateral sclerosis (ALS), multiple sclerosis, diabetes, rheumatoid arthritis and cancer... Notably, many of these diseases are age-related, but ROS reaction products increase during normal aging too. Notably, there is a lack of definitive evidence on the contribution and/or association of excessive ROS generation to/with age-related diseases, which is in no way helped by shortcomings in utilized/available biomarkers and deficiencies in analytical methods for accurate and reliable analysis.
There is no doubt that ROS can exert major damages to cellular components and biomolecules such as biological membranes, DNA, proteins and lipids. Oxidative protein modifications may induce loss of functional integrity, leading to inhibition of enzymatic and binding activity, susceptibility to aggregation and proteolysis, altered uptake by cells, and increased immunogenicity.
ROS may also oxidize membrane lipids and can impair membrane function due to altering fluidity and inactivating integrated membrane-receptors. In particular, polyunsaturated fatty acids (PUFA) are susceptible to attack by ROS, which may lead to enhanced levels of oxidized phospholipids (OxPLs) and exhibit pro-inflammatory and pro-atherogenic properties.
In short, lipid oxidation products are interesting because they are biomarkers of oxidative stress – and OxPLs (because of their roles in membrane function and distinct signaling cascades) are particularly important. Overall, the available information on bioactivity of various OxPLs is limited, and their utility as biomarkers is hindered three-fold by their structural complexity, the scarce availability of published analytical methods, and the limited sensitivity of methods (we are looking for trace amounts in the presence of huge excesses of non-oxidized PLs). Which OxPLs should be part of a powerful biomarker panel? Only adequate analytical methods hold the key to answering that question.
It is not surprising that stereoisomer separations caught our attention. If, for example, ROS attack a PUFA with two double bonds, such as linoleic acid, a single preferential methylene position for preferential attack is available, which results in stabilization into two possible preferential constitutional isomers (regioisomers). Because a new stereogenic center is formed by oxidation, a racemic mixture is formed as there is no stereogenic preference for non-enzymatic oxidation. Therefore, a single compound is transformed into four isomers. If this happens, for example, on a PL with a single linoleic acid, the chirality of the sn2-position of the glycerophospholipid means that the enantiomers change into diastereomers (or epimers). Since RPLC can resolve diastereomers, up to four peaks could become visible in the RPLC chromatogram. If a PL with single arachidonic acid side chain has three methylene groups that are attacked by ROS, it gives six preferential positions for the formation of primary oxidation products, for example, hydroperoxide. Because of sn2 chirality, 12 diastereoomers are present! Moreover, primary oxidation products of PUFA are accompanied by further oxidative degradation (cyclization, side chain fragmentation, transformation to other functional groups), so the actual number of oxidation products isn’t clear. You get the picture: there is an avalanche of possible OxPLs, and it is unclear which of them is bioactive and of practical relevance.
To nail down this structural complexity of PUFA oxidation to a specific example, consider isoprostanes, which are metabolites of free radical-catalyzed arachidonic acid peroxidation. Up to 64 distinct structures (four regioisomers each in 16 stereoisomeric forms) of four major structural classes (H2-, F2-, D2-, E2-isoprostanes) may be formed by this non-enzymatic arachidonic acid peroxidation process (note, minor isoprostanes and other follow up reaction products of H2-isoprostanes are not even considered). Among them, 8-iso-prostaglandin F2α has attracted particular attention due to its biological activity as a potent vasoconstrictor in lung and kidney tissue. A lack of research means that the biological activities of other isomers are unknown.
The enormous structural complexity may be the reason that simple structures such as malondialdehyde (MDA) and 4-hydroxynonenal (HNE) are often analyzed as biomarkers of oxidative stress instead of OxPLs. However, (various) OxPLs may be bioactive and interfere with signaling pathways downstream of membrane processes. Our goal is, therefore, to analyze the structural complexity in a more comprehensive way using selective chromatography hyphenated with high-resolution mass-spectrometry and selective MS/MS transitions that implement MS/MSall with Sequential Windowed Acquisition of All Theoretical Fragment Ion Mass Spectra (SWATH) technology. Ion-mobility analyzers hyphenated with such LC-MS systems are further strategies to cope with the enormous diversity of structural isomerism and complexity. Nanoparticle-based sample preparation and enrichment platforms help us to reach adequate sensitivities, which was my presentation topic at HPLC 2015 in Geneva last month.
Overall, the project is very challenging but also absolutely fascinating – in my opinion, much more research is needed; it will certainly keep our group busy for quite a while.
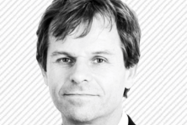
Michael Lämmerhofer is a Professor at the Institute of Pharmaceutical Sciences, University of Tübingen, Germany