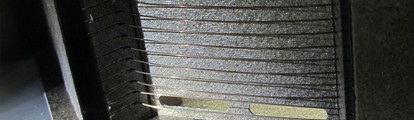
Faster Chemical Separations
A 2015 Innovation Award-winning “fastGC” module enhances proton transfer reaction time-of-flight analyzer performance by adding an optional near real-time chemical pre-separation step. Here’s the story behind its development.
Lukas Märk |
The Problem
Although gas chromatography (GC) offers chemical separation and identification, it can take a lot of time to perform the experiment and analyze the results when compared with real-time gas analyzers, which provide dynamic information about a process. Is it possible to combine the key benefits of both technologies?
Background
With efficient, soft ionization and high mass resolution, proton transfer reaction time-of-flight (PTR-TOF) analyzers have become the de-facto standard for real-time monitoring of trace volatile organic compounds (VOC). In a fraction of a second, a large spectrum of VOCs can be analyzed right down to parts per trillion (ppt) levels. Such high performance is ideal for monitoring complex samples in a variety of applications, including environmental monitoring, food and flavor research, life science applications, industrial process monitoring and many others. With a high-resolution TOF mass spectrometer, isobaric compounds can easily be identified by their difference in exact mass. However, isomers (compounds with the same chemical composition and consequently the same exact mass) are not separated in a TOF. Using GC pre-separation in combination with a PTR-TOF resolves this problem, but at the expense of real-time analysis, since a typical GC run takes around 30 minutes – more than a thousand times longer than a PTR-TOF analysis.
The run time of a GC begins at sample injection and finishes when the final compound elutes from the column, which depends on several factors – most importantly the column temperature. To speed up the process the column is heated (up to several hundred degrees), typically in an oven that represents the most significant contributor to the thermal mass of the system, which limits the speed of heating and also cooling. This is improved in fast-GC systems, which, in most approaches, reduce the thermal mass of the system (for example, by combining the column directly with a heating wire). Such systems reduce the runtime down to several minutes. A higher heating rate is usually achieved by applying more power – but a fast cooling rate is more difficult to accomplish. Of course, the cooling time sets a limit on when the next analysis can take place and therefore restricts the throughput.
Although combining a PTR-TOF with a GC pre-separation is tempting to get the additional chemical dimension, the trade-off in time resolution is often too big a sacrifice, even at only a few minutes. The IONICON fastGC is designed specifically as an add-on for PTR-TOF trace gas analyzers to enable another dimension of chemical separation, mainly for separation of isomers and enhanced identification of compounds. The objective was to get very short run times, so that the real-time capabilities of our fast analyzers were sacrificed as little as possible.
The Solution
The fastGC system uses an innovative heating concept that allows much faster heating rates –more than 30K/s compared with 30 K/min in conventional GC systems. Most importantly, it also offers cooling rates of 30 K/s, which greatly increases the overall sample throughput.
For our fastGC, we use a commercially available metallic GC column, but trim its length to a few meters. By applying an electric current directly to the metallic column, it acts as its own heating wire. Here, we approach the lowest possible thermal mass, with only the column itself changing temperature and a resultant rapid heating and cooling response. Our concept demanded precisely controlled heating and the ability to regulate power so that the target temperature could be reached as quickly as possible. In essence, we needed to meet two major challenges preferably with a single solution. The first challenge is the regulation of the heating power; with an overall low resistance, a high current must be applied and regulated. The second (perhaps less obvious) problem is the measurement of the column temperature. In simple terms, a temperature sensor attached to the thin column would likely result in the column having the temperature of the sensor and not the other way around...
To resolve the first problem – controlling the heating power – we used pulse width modulation. Fast electrical pulses (several kHz) at variable lengths are applied to the column. The on-off duty cycle defines the average heating power. The voltage is constant, but the resistance of the column, which changes (slightly) with temperature, determines the resulting current. This also led to the solution of the second problem – the temperature measurement. Comparing the attained current to the voltage, we can calculate the column resistance, and from this the column temperature. To read the temperature in the absence of heating, we limit the minimum duty cycle to a pulse just long enough to measure the temperature correctly but resulting in negligible heating.
By placing the column in an oven, we can calibrate its resistance to temperature response. Since the change in column resistance with temperature is small, drifts and other resistances can affect the measurements. Therefore, we zero the temperature measurement at regular intervals, especially after start-up. We do this by cooling the column for an extended time to ensure that its temperature is at ambient. An independent sensor measures the ambient temperature. A built-in microprocessor determines and subtracts the offset resistance; the microprocessor also controls the heating power to quickly reach a set temperature or drive temperature ramps.
The column can be heated to several hundred degrees, limited by the maximum allowed column temperature. The column is fixed to a special mount, which is resistant to high temperatures, is non-conductive, and has a minimal contact with the column, as shown in Figure 1.
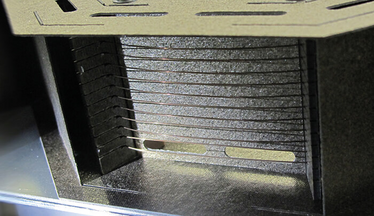
Figure 1. IONICON fastGC system, column and mount.
The whole setup is placed inside a housing. Air openings and a fan allow for a controlled cooling of the column with ambient air. Due to the low thermal mass, this allows cooling rates of 30K/s. Mounting it next to a PTR-TOF allows access to the ambient air for cooling on one side, while the other is in direct, cold-spot free contact with the heated inlet chamber, where the sampling gas arrives through capillaries. For real-time trace gas analysis, the use of heated, inert wetted-surfaces is important to reduce surface interaction and retention effects.
We use an array of several micro-valves to configure the gas flow. A sampling loop (an inert capillary with an internal volume of several ml) draws the sample gas constantly. To initiate a fastGC analysis, gas from the sampling loop is injected into the column. By controlling the flow and the time, the amount injected can be varied. A digital mass flow controller regulates the flow of clean N2 carrier gas to push the sample through the column. When a lower flow is set than needed for normal PTR-TOF operation, additional N2 is used as make-up gas. The valves can also be configured to bypass the fastGC setup and perform normal real-time analysis with the PTR-TOF, which allows real-time monitoring with (automated) intermediate fastGC analyses.
Beyond the solution
The fastGC is available as a built-in option for our ultra-sensitive, real-time PTR-TOF analyzers, but can also be used to upgrade existing PTR-TOF systems. The key feature of the fastGC – adding a dimension of chemical separation to PTR-TOF data – has already been demonstrated in several publications; for example, the separation of several (isomeric) monoterpenes in biological samples (1). Monoterpenes are a group of biological VOCs emitted in high quantities by numerous plant species, particularly conifers and are associated with the typical scent in a forest. A PTR-TOF can record the combined contribution of these isomers, and – with the addition of the fastGC – the contributions of different monoterpenes can be monitored individually (see Figure 2 for another example).
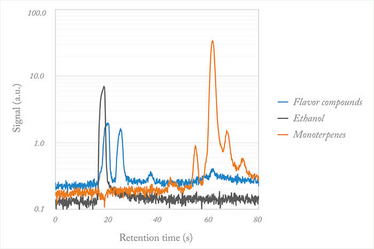
Figure 2. FastGC-PTR-TOF head-space measurement of a beer-lemonade mixture. Ethanol has eluted from the column before other flavor compounds and does not perturb their measurement. At least five different monoterpenes, with a dominant contribution from limonene, can be separated.
Additional benefits of the fastGC add-on quickly became apparent in other applications, such as in the headspace analysis of alcoholic beverages, where the high ethanol concentration has so far compromised the measurement of other trace VOCs. Using the fastGC system, the ethanol quickly elutes from the column and subsequently does not interfere with the measurement (2). An example is shown in figure 2. This principle can be applied to other scenarios with high matrix concentrations.
The high rates for heating and cooling surpass those of other commercially available fast GC systems. And with minimal effort, the IONICON fastGC could eventually be adapted for integration with other gas analyzers. The swift acceptance and a rapid spread of the fastGC add-on that we have seen in PTR-MS user groups demonstrates great potential – and we expect to see its use spreading towards routine applications. Once established, we feel fastGC-PTR-TOF could go as far as replacing conventional GC-MS because the initially greater costs are balanced by higher throughput.
Currently, we are working on fully integrating the fastGC-PTR-TOF instrument with an auto-sampler set-up (soon to be launched for the IONICON PTR-MS products). Our ultimate goal is to develop an automated instrument that includes all components from sampling to chemical pre-separation to PTR-TOF analysis, followed by appropriate data processing – a challenge we are excited to tackle.
The Road to fastGC
1995: The first PTR-QMS (quadrupole MS) was developed
1998: IONICON founded as a spin-off of the University of Innsbruck, Austria
2007: The first PTR-TOFMS (time of flight MS) was launched
2013: fastGC development started
2014: Launch of fastGC
2015: fastGC first presented to the public at Pittcon 2015; fastGC receives “Golden Gas Award 2015” from Gases & Instrumentation International; fastGC receives “Innovation Award” from The Analytical Scientist.
- D Materić et al., “Monoterpene separation by coupling proton transfer reaction time-of-flight mass spectrometry with fastGC”, Anal Bioanal Chem, 407(25), 7757–7763 (2015). PMID: 26253230.
- A Romano et al., “Wine analysis by FastGC proton-transfer reaction-time-of-flight-mass spectrometry”, Int J of Mass Spectrom, 369, 81–86 (2014).
CEO, IONICON Analytik GmbH, Innsbruck, Austria.