Gurus of the monolith
Four stationary phase experts – Emily Hilder, Frantisek Svec, Nobuo Tanaka and Sebastiaan Eeltink – discuss the unfolding story of monolithic columns: from chromatographic curiosity to the future of sample preparation?
Emily Hilder, Sebastiaan Eeltink, Frantisek Svec, Nobuo Tanaka |
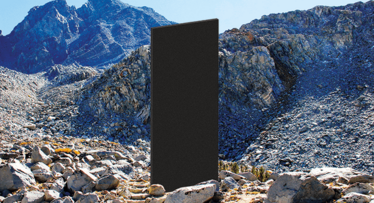
Nobuo Tanaka
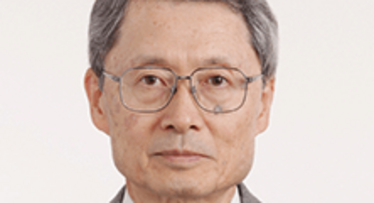
Nobuo Tanaka was educated at Kyoto University in Japan. After graduation, he spent several years as a postdoctoral researcher at University of Pennsylvania, University of Washington in Seattle, and finally Northeastern University in Boston. He returned to Japan in 1979 and worked his way up to full professor at Kyoto Institute of Technology where he was active for 30 years until his retirement in 2009. Since then, he has been a technical advisor at GL Sciences. Tanaka’s most important contribution was the development and reduction to practice of monolithic silica columns for HPLC. Now, he contributes to the development of high-speed, high-efficiency monolithic silica columns and the operation methods that can enhance the performance of HPLC.
Frantisek Svec
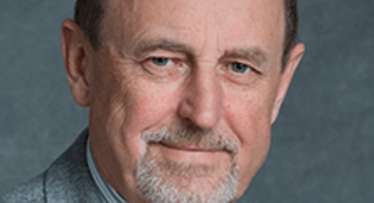
Frantisek Svec is currently professor at Beijing University of Chemical Technology and PI in the International Centre for Soft Matter there. He worked for the Academy of Sciences in Prague for several years before he joined faculty first at Cornell University, and then at the University of California-Berkeley and the Lawrence Berkeley National Laboratory. Svec received numerous honours for his world-leading contribution to chromatographic science, including the ACS Award in Chromatography and the Martin Medal, and is recognized for his extraordinary work in developing polymeric stationary phases and their adaption to multiple column and chip formats. His pioneering developments have been adopted widely by numerous commercial organisations. Svec is editor-in-chief of the Journal of Separation Science and is a member of the editorial boards for several leading chromatographic journals.
Emily Hilder
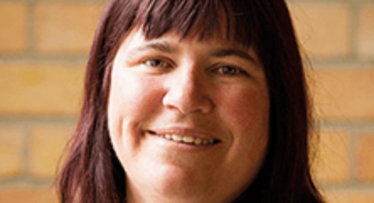
Emily Hilder is a graduate of the University of Tasmania, awarded her BSc(hons) in 1997 and PhD in 2001. She held postdoctoral positions at Johannes Kepler University (Austria) and the E.O. Lawrence Berkeley National Laboratory and University of California, Berkeley (USA). In 2004 she joined the Australian Centre for Research on Separation Science (ACROSS) at the University of Tasmania where she held an ARC Australian Postdoctoral Fellowship from 2004-2007 and ARC Future Fellowship from 2010-2014 and was promoted to full Professor in 2011. She is currently Head of Chemistry and Director of the ARC Training Centre for Portable Analytical Separation Technologies (ASTech) at the University of Tasmania. Her research focuses on the design and application of new polymeric materials to improve analytical separations and on approaches to make analytical systems smaller and more portable. She is an Editor of the Journal of Separation Science and a member of the Editorial Board for a number of other journals.
Sebastiaan Eeltink
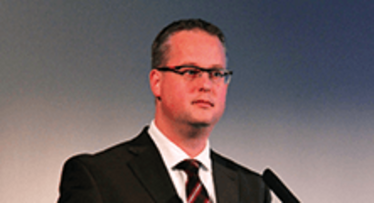
Sebastiaan Eeltink received his PhD degree in chemistry (specializing in analytical chemistry) in 2005 from the University of Amsterdam. Thereafter, he conducted postdoctoral research at the University of California, Berkeley, USA, and was guest scientist at the Lawrence Berkeley National Laboratory. In 2007, he joined Dionex and conducted research on packed and monolith column technology for ultra-high-pressure LC, two-dimensional LC, and nanoLC. Eeltink is now research professor at the Department of Chemical Engineering at the Free University of Brussels, where he focuses on the development, characterization, and application of novel chromatography materials, including nano-structured monolithic materials and coatings in capillaries and micro-fluidic devices, for ultra-high-pressure and multi-dimensional (spatial) LC-MS separations.
Monoliths in a Nutshell
By Nobuo Tanaka, GL Sciences, Tokyo, Japan.
My group is working on a whole range of high-performance monolithic silica columns as well as sample pre-treatment devices. Personally, I am interested in evaluating small, high-speed, high-efficiency monolithic silica columns in addition to finding new applications and optimization methods. Here, I offer a snapshot of the world of monolithic (silica) columns.
Unique qualities
- Provide the efficiency of UHPLC while lowering the pressure in HPLC.
- Long rod-type monolithic silica columns can provide 100,000 theoretical plates for high-resolution applications.
- Long monolithic silica capillary columns (several meters in length) generate several hundred thousand theoretical plates in a single column. Connecting columns together can generate one million theoretical plates with practical pressure and time improvements.
- Allow very fast purification of antibodies (seconds rather than minutes using particulate columns).
Key milestones
- Silica monolith preparation using the sol-gel method.
- Successful cladding with PEEK (polyetheretherketone) – biocompatible, chemically inert to most solvents, and can be used to replace stainless steel tubing.
- Capillary monolith preparation.
- Long, high-efficiency capillary columns.
- Development of second-generation monolithic silica, with reduced-size through-pores and skeletons and reduced external porosity.
Analytical role
- Faster separations at fixed pressures.
- Lower pressures for similar resolutions.
- Low-pressure sample pretreatment device, utilizing high permeability.
- Ultra-high resolution using very long capillary columns.
- Low-pressure, very fast purification of antibodies.
Success stories
- One-shot RPLC proteomics (identification of more than 2600 proteins with one shot onto meter-long columns).
- One-shot hydrophilic interaction liquid chromatography (HILIC) proteomics with meter-long HILIC (capillary) column (orthogonal proteomics together with reversed phase [RP]).
- Demonstrate resolution based on the difference of one H/D (hydrogen/deuterium).
- High-permeability solid-phase extraction (SPE) cartridges (that are spun to enable elution) for general and DNA extraction purposes.
- Protein purification by a protein-A or protein-G immobilized column; much faster purification of antibodies than by particulate columns.
Big players
- Kazuki Nakanishi, Kyoto University – the original inventor of monolithic silica, leading the development of new monolithic materials for separation science.
- Hiroyoshi Minakuchi, Kyoto Monotech – a co-worker in the development of monolithic silicas, commercialization of various monolithic silica products.
- Karin Cabrera, Merck – the leader in the first successful commercialization of PEEK-clad monolithic silica column (Chromolith), who went onto develop the second-generation of the technology.
- Takeshi Hara, Free University of Brussels – developed a second-generation monolithic silica column.
- Merck produces Chromolith (first- and second-generation monolithic silica rod columns).
- GL Sciences, Inc. provides a wide range of monolithic silica products, including polymer-clad, glass-clad monolithic silica columns (MonoTower, MonoClad), monolithic silica capillary columns (MonoCap) as well as an SPE-devices for small molecules (MonoSpin) and DNA (MonoFas).
- Kyoto Monotech focuses on the development of various monolithic silica products, including those for HPLC and antibody separations.
Hot innovation
- Short-small-diameter column (1 mm ID, 5 cm), high-speed, high-efficiency columns for LC-MS.
- Emergence of a comprehensive range of analytical HPLC columns, 0.05-4.6 mm ID: 1–50 cm long for rod columns, and 5–200 cm long for capillary columns.
- High-performance columns with sub-50 micron ID – 4.6 mm ID showing performance comparable with current UHPLC.
- Long chiral stationary phases on monolithic silica capillary columns.
- The development of an on-line sample treatment device utilizing high permeability.
- Columns that can be eluted by spinning to achieve fast purification of antibodies within minutes.
- Ninety six-well plates with monolithic silica columns at the bottom of each well for automated antibody purification.
Future challenges
- Further reduction of structural features to attain the performance of a column packed with sub-1.5 micron particles.
- Reproducibility in monolith preparation and on-column modification (in capillary), high-efficiency products for HILIC, ion exchange, and chiral chromatography.
- Preparation in silica-coated stainless steel tubing.
- The development of a separation device for particulate matters, including very large molecules and cells.
- Large and small columns, and tubular columns (elution in the radial direction) for high-throughput (large-scale) purification of biopharmaceuticals.
- Disposable column for antibody purification.
Bold predictions
- Monoliths could dominate the small-size column market, particularly capillary columns, and, especially long columns, for LC-MS, for proteomics and metabolomics (separation of large numbers of components, high resolution separation).
- Monoliths could dominate the field of (high-speed) antibody purification.
Images courtesy of Dario Arrua, David Schaller and Wei Boon (Jason) Hon from ACROSS, University of Tasmania, and Karsten Goemann from our Central Science Laboratory, also at the University of Tasmania.
How would you describe the importance of monoliths?
Frantisek Svec: I’ll cite the late Georges Guiochon from over 10 years ago: “The invention and development of monolithic columns is a major technological change in column technology, indeed the first original breakthrough to have occurred in this area since Tswett invented chromatography, a century ago.” (1)
I would say monoliths are among the five most important chromatography developments, which I would rank as:
- Development of HPLC using bonded porous silica particles.
- Introduction of ion exchange chromatography for the separation of proteins.
- Improvements in capillary electrophoresis enabling rapid sequencing of nucleic acids and deciphering the human genome.
- Advent of proteomics and metabolomics.
- Novel formats of separation media, including monoliths, sub-2 micrometer packings, and core-shell particles.
Emily Hilder: Monoliths were the first practical alternative to particle-based stationary phases in chromatography. Both silica and polymer monoliths were able to demonstrate fast separations without significant loss in separation efficiency. Irrespective of whether or not monoliths outperformed other column types, the development of this new column format triggered many other innovations in separation science. Monolithic columns quickly became pervasive (particularly polymer monoliths) as many researchers could easily and cheaply make them in their own lab.
Sebastiaan Eeltink: Silica- and polymer-monolithic columns are yet to breakthrough into industry, so I feel their direct impact in separation science is rather limited. Although the gold standard for separations is the packed column, monoliths have the intrinsic potential to perform better because porosity and globule/macropore size can be tuned. To increase the separation power of LC with at least one order of magnitude, new routes should be explored, and I believe that monoliths can ultimately deliver an advantage.
What would you consider the most important development milestones?
FS: The idea that a continuous piece of porous material can be used as a separation medium was not new. It was postulated as a theoretical option by Robert Synge more than 60 years ago (2). However, he could not find any materials that would enable flow through separation without collapsing. Kubin’s first experimental demonstration that included a hydrogel-based monolith was not very successful either because of poor permeability (3). Better results were achieved later with polyurethane foams prepared within the confines of the column that partially separated both in GC and LC modes (4, 5).
Stellan Hjertén prepared a monolith-like structure by compressing irregular pieces of a crosslinked polyacrylamide gel to form a continuous bed and demonstrated rapid separations of proteins using ion exchange chromatography (6). The first genuine porous polymer monolith was the disc we prepared by bulk polymerization of methacrylate monomers, which enabled rapid separation of proteins using various mechanisms (7). We then also prepared the first highly permeable methacrylate-based columns and demonstrated separation of proteins in seconds using a high flow rate (8). Finally, Nobuo Tanaka published fast separation of small molecules using C18 silica-based monolith (9). These three “new era” monoliths were then commercialized.
SE: For me, the high-speed separation of five proteins within one minute on a polymer monolithic column by Frantisek Svec is notable. Also Nobuo Tanaka performed a feasibility study with silica monoliths to achieve better efficiency and better permeability with respect to packed columns. There’s also Christian Huber’s work in high efficiency liquid chromatography-mass spectrometry (LC-MS) DNA separations.
EH: In brief, I would say the first development of polymer (Svec and Frechet) and silica (Nakanishi, Minakuchi, and Tanaka) monoliths in the 1990s – and the first commercial embodiments (Merck – silica monoliths, BIA Separations, Dionex – polymer monoliths).
Have monoliths replaced other column materials in certain applications?
FS: Monoliths are not a replacement. They are complementary to other materials used for chromatographic separations. Their emergence has not lead to any significant change in the use of packed columns. However, the enhanced speed of separations using monoliths renewed interest within the chromatographic column industry for developing technologies that reduce the effect of diffusion on separations in packed columns. As a result, sub-2 µm and core-shell particles emerged that enable accelerated separations in packed columns.
EH: I agree with Frantisek. Replace is a strong word, and not one I would use in describing the impact of any column format in separation science. I do believe they can outperform other materials in certain circumstances, but I am not convinced that they are an absolute replacement.
Where fast, generally lower resolution separations are required, monoliths can be preferable to other column types. For example, they are often used for matrix removal for targeted analysis of drugs and metabolites in biological matrices with mass spectrometry (MS) detection. Polymer monoliths outperform particle packed columns for many protein separations, including very large proteins, such as monoclonal antibodies.
They are also very rugged columns that exhibit extremely high pH and temperature stability. Indeed, polymer monoliths are one of the few stationary phases that can be used at very high temperatures (up to 300 °C), and unlike other temperature stable materials, they are much more inert and do not promote catalytic degradation of analytes, which means that even proteins can be separated at temperatures up to 130 °C.
SE: Currently, monolithic materials – especially polymer monoliths – are only (slowly) replacing packed columns for large biomolecule separations. Although there is a lot of potential with functional monolithic materials (for example, enzymatic reactors) for a wide range of applications, I would argue that similar results may be achieved using functionalized particulate materials.
So, what role have monoliths played in separation science?
FS: Monoliths were initially a curiosity to chromatographers. The explosion in use of monolithic materials followed the renewed interest in capillary electrochromatography (CEC) in the mid 1990s, because preparing monoliths in capillaries was easy and avoided the need for frits. A wide variety of monolithic approaches have been developed and successfully applied for efficient separations in CEC. The development of chromatographic nanoflow hardware and popular application of MS underlined the need for capillary columns in LC. Here again, monoliths in columnar and porous layer open tubular (PLOT) formats play an important role.
Monoliths were also the first technology to enable very fast chromatographic separations. In particular, this was beneficial for large molecules, where separations were previously slow. Let’s cite Georges Guiochon again (10): “The recent invention and development of the monolithic columns is a major technological change in column technology. This new process of manufacturing columns holds great promises […] Despite the technological and economical difficulties, the monolithic columns will eventually prevail because their principle provides a systematic approach to modify and optimize separately the sizes of the different geometrical elements necessary to do chromatographic separations, the through pores, the porons, the domains, and the mesopores.”
EH: As I intimated earlier, the introduction of monoliths as a very different type of stationary phase format has contributed to (and possibly catalyzed) a great deal of work on stationary phase design and also theoretical studies on what an ideal stationary phase should look like. Exploring different formats is important for answering these questions and, for me, the impact of monolithic materials will remain significant because of the role they have played in asking and answering some of these questions.
As easily prepared porous materials, the applications have extended beyond separation science. This is particularly the case for polymer monoliths, which can be very easily prepared in a range of formats. For example, polymer monoliths prepared in flat sheets have been used for thin layer chromatography (TLC) but also as substrates for matrix-assisted laser desorption ionization (MALDI) MS or as an alternative to paper for paperspray MS. They’ve also been used in dried blood spot sampling and for the formation of superhydrophobic films with exciting properties such as complete water repellency, self-cleaning, separation of oil and water, and antibiofouling.
SE: The development of monolithic structures is one of the routes to improve separation efficiency in chromatography.
Several groups have developed novel synthesis routes and have also characterized their performance. As such, the development contributed to the general understanding of the effect of morphology on band broadening. These new developments have also enabled new applications, especially in the bioanalysis field.
What have monoliths made possible in a broader sense?
EH: Monoliths are much better suited for miniaturization than packed column formats because they don’t suffer the same challenges that come with trying to pack particles into very narrow bore tubing. Micro and nano-scale columns are much more compatible with MS detection, and result in much better detection sensitivity. Micro and nano-scale monolithic columns have had a major impact in peptide and protein analysis by LC-MS for proteomics applications.
SE: I think the analysis of biomolecules (large proteins, antibodies), although there are examples that demonstrate that these separations can also be performed on columns packed with silica particles (but, they are not as efficient).
Can you tell us a bit about how you and your group are using monoliths?
EH: I’ve been working with monoliths for about 14 years now. My earlier contributions demonstrated new approaches to functionalize monolithic materials, in particular approaches using UV light, such as photografting. At that time we also were able to demonstrate the advantages of using monoliths for capillary electrochromatography. I was one of the first to demonstrate the advantages of adding nanoparticles to monolithic materials. This approach has since been followed by many others, including being using in commercial columns. My group continues to work with nanoparticles and monoliths but our focus has moved away from trying to change the functionality, to trying to change the structure to produce more ordered materials. Nanoparticles can help with this, and we are also focusing on new synthetic approaches including cryopolymerization and other templating approaches.
My group is also focusing on demonstrating new applications for polymer monoliths, in particular for sampling and sample preparation where these materials can offer significant advantages. We’ve developed a flat sheet format that can be used instead of paper for dried blood spot sampling (MilliSpot) or paperspray MS, as well as materials in other formats such as pipette tips, which we are able to use in miniaturized formats for sample storage, preparation and analysis of biological fluids such as whole blood, plasma and urine.
SE: My research aims at the development, characterization, and application of novel chromatography materials, including nano-structured monolithic materials in capillaries and micro-fluidic devices. The novel technology is applied to establish ultra-high-pressure and multi-dimensional (spatial) LC-MS separations of complex life-science mixtures.
Who are the pioneers in monolith development and what was their role?
EH: Frantisek Svec, Stellan Hjerten and Tatiana Tennikova all made seminal contributions to the development of polymer monoliths. Svec (together with Frechet) introduced rigid macroporous monoliths for analytical separations, with the same approach used by a majority of researchers today. Tennikova was involved in the early development of monolithic materials for purification of biological molecules. At a similar time, Hjerten introduced softer materials based on polyacrylamide.
Nobuo Tanaka introduced silica monoliths as we know them and together with others, in particular Kazuki Nakanishi has continued to drive innovation in this area.
Silica monoliths have been a commercial success because of the significant research and development by Merck (Chromolith) and more recently GL Sciences.
Similarly, polymer monoliths have been successfully commercialised by BIA Separations in disk formats (CIM – convective interaction media) and in preparative scale for purification of biomolecules. Dionex (now Thermo Scientific) were the first company to demonstrate reproducible production of analytical scale monolithic columns (ProSwift range).
All of these companies have made very considerable contributions to the field through their research and development programs.
FS: I’ve already mentioned most of the great scientists. I would like to mention one more; one who remains somewhat forgotten, the late Russian professor Boris Belenkii. He developed a theory of short separation layers for chromatography of large molecules in the late 1980s. However, millimetre thin layers were difficult to prepare from particles due to the massive channeling and he was searching for material that would enable validation of his theory. His tireless efforts and collaboration with my group in Prague then led to the development of monolithic discs. This technology was then put into production by BIA Separations in Slovenia in the early 1990s. Based on the success of the discs, this company continued developing the technology and invented highly innovative monolith formats: tubes that are used in columns that have a radial flow pattern. This technology allowed scale up of the monolithic columns to volumes up to 8 L, which enabled their application in the biotechnology industry.
Thermo Fisher Scientific (formerly Dionex) manufactures monolithic columns in a wide variety of chemistries and sizes specifically designed for the separations of large molecules using reverse phase, ion exchange, hydrophobic interaction, and affinity mechanisms.
Silica-based inorganic monoliths are produced by Merck in Germany and Phenomenex in California. The commercialization process required development of a new technology for the preparation of columns. This is because the silica monoliths are prepared initially as self-standing rods that must be clad with a polymer tube to create the desired column.
SE: I believe that the separation field broadly recognizes two monolith heroes: Tanaka (silica monoliths) and Svec (polymer monoliths). Interestingly, they both focussed on completely different areas. Tanaka recognized (and demonstrated) that monolithic stationary phases have the potential to perform intrinsically better than packed columns, where the efficiency is related to particle size but the total porosity is fixed. By tuning the morphology of silica monoliths Tanaka created separation structures that outperform packed columns. Svec is an outstanding polymer chemist and he demonstrated the potential of polymer monolithic materials for a broad range of applications by creating really unique surface chemistries.
How do you see monoliths being developed in the future?
EH: Monoliths are already very important for miniaturized separation systems as they are easily fabricated in narrow bore tubing or within microfluidic devices. I anticipate that monoliths will continue to play an important role in miniaturization. The excellent flow properties and ease of synthesis in a range of formats make monoliths well suited for sample preparation, such as for solid phase extraction. These features, combined with the drive towards small volume sampling and miniaturized sample preparation mean that we should expect monoliths to play a very significant role in sample preparation methods in the future.
Looking beyond analytical science, there are many other porous polymer or ceramic monolithic structures that have been developed for other applications, and some which offer more ordered structures that could be better suited for high performance chromatography. I see incredible opportunities to apply new types of monolithic structures for analytical applications, and also in the development of new types of monolithic structures.
FS: As noted by Emily, I expect to see further developments in miniaturized separation devices equipped with monoliths. In particular, monoliths may find numerous applications in capillary techniques because packing efficient capillary columns with particles remains difficult. Several groups are also testing new polymerization mechanisms and chemistries that can make the preparation of monoliths even easier. As polymer chemistry offers a lot of options, this trend will continue.
It is also possible that new developments will result in new morphologies – at least in the area of polymer-based monoliths. I expect these studies to lead to organic polymer monoliths that will be easy to prepare, yet enable fast and highly efficient separation of small molecules in the isocratic mode. I also foresee significant extension of monoliths in the fields of GC and thin layer chromatography (TLC). The initial studies indicate that monoliths may represent a new quality in these formats. With the increased interest shown by the biopharmaceutical industry in purification and separation of very large molecules and particles such as antibodies, viruses, and even microbial cells, I expect that the commercial use of monolithic devices will grow because monoliths are best suited for this task.
SE: Morphology optimization is a key aspect (as is the need to tune the macropore and globule size). Polymer monoliths have a lot of potential for the separation of peptides, intact proteins (including protein isoforms), and even large biopharmaceutical molecules, such as antibodies. The real potential (good efficiency, low carry-over, analyzing large molecules and also samples in “dirty” matrices, such as fermentations) for these applications has not yet been fully demonstrated.
Recently, the pressure stability of polymer monolithic stationary phases (with optimized pore structure) in capillary column formats has been demonstrated at 80 MPa. Using 50 mm short gradient separations of simple peptide and protein mixtures can be realized within sub-minute gradients. Therefore, it is very attractive (in terms of peak-production rate) to use this technology as the second-dimension column in a two-dimensional set-up.
Monolithic materials are prepared from liquid precursors. After filling, within the confines of microfluidics devices, the stationary phases can be created at the desired location by applying, for example, a UV initiated polymerization approach. Especially when advanced channel designs are created on chip (for example, parallel separation channels) packing with particles is almost not an option. This is one of the unique selling points of monolithic stationary phases.
I am also sure that with 3D printing technology developing fast, it may soon be possible to design highly homogenous monolithic structures, with optimized (nano) feature sizes.
What challenges lie ahead?
EH: Both a strength and a weakness of this technology is the ease of synthesis of monoliths. Most researchers that use monoliths have their own recipes and most materials are very poorly characterized. With the synthesis of classical polymer monoliths now well understood, the challenge is to demonstrate applications where these materials offer advantages over existing materials. For example, the exceptional temperature and pH stability of polymer monoliths means they can be used for high temperature chromatography (up to ~250 °C). Exploring new synthetic approaches to access new types of morphology will also be important for future developments in this field.
As with other technologies, future developments will also depend on a wider range of companies developing new products based on monoliths.
SE: To really exploit high-porosity monolithic supports at a practically relevant range of analysis times, the macropore and globule size needs to be carefully tuned (that is, decreased), while the structural homogeneity must be maintained. Hence the development of silica and polymeric nanostructures could be an interesting upcoming development.
I believe that the pressure stability of silica monolithic materials may constitute a problem because chromatographic performance limits are related to the maximum allowable pressure in terms of efficiency and analysis time.
Although there are examples in literature of polymer monoliths yielding high efficiency separations of small molecules (as demonstrated by Gasparini’s group), polymer monolithic columns generally perform poorly for small-molecule separations. This is not fully understood, but it is likely to be related to surface diffusion effects. So, we need to improve our knowledge on dispersion.
Where will monoliths be in 10 years?
FS: I believe the future for monoliths is bright. They are likely to be used extensively in sample preparation, a rapidly developing area. I also expect a renewed interest in TLC using ultrathin monolithic layers. TLC is a very simple and inexpensive technique directly compatible with MS. Novel concepts of two-dimensional and three-dimensional (3D) liquid chromatography using 3D monolithic cube-like structures have been suggested recently. Although convincing results have yet to be demonstrated, the concept itself is very interesting and once tuned, it may represent a significant future breakthrough.
Another area where I expect to see new and exciting developments is in 3D printing of porous polymer monoliths and complete devices as noted by Sebastiaan. Their exact morphological structure and shape can be designed using software and their exact replicas can then be reproducibly printed. A computer-aided design approach is likely to enable industrial mass production of identical chromatography columns with performance superior to those now in use.
EH: Within the next 10 years I expect monoliths to be viewed as a mature technology, with more commercial products available and for a wider range of applications.
SE: I hope that the potential will be realized and that polymer monolithic columns become a choice technology for establishing high-efficiency LC-MS and multi-dimensional biomolecule separations.
I believe the technology provides unique profiling possibilities for complex proteomics mixtures encountered in biomarker discovery studies, and also for biotech mixtures (for example, fermentation). Hence column technology has the potential to contribute (when applied in advanced separation workflows) to our understanding of disease pathways, to the development of novel therapy regimes, and to greatly improved biotechnological processes.
- M. Al-Bokari, D. Cherrak D, and G. Guiochon, “Determination of the Porosities of Monolithic Columns by Inverse Size-Exclusion Chromatography.” J. Chromatogr. A., 975(2), 275-84 (2002).
- D. L. Mould and R. L. M. Synge, “Electrokinetic Ultrafiltration Analysis of Polysaccharides. A New Approach to the Chromatography of Large Molecules”, Analyst, 77, 964-969 (1952).
- M. Kubin, P. Spaéek, and R. Chromecek, Collect. Czech. Chem. Commun., 32, 3881, (1967).
- T. R. Lynn, D. C. Rushneck, and A. R. Cooper, J. Chromatogr. Sci., 12, 76–79 (1974).
- L.C. Hansen and R. E. Sievers, J. Chromatogr., 99, 123–133 (1974).
- S. Hjertén, J. Liao, and R. Zhang, “High-performance Liquid Chromatography on Continuous Polymer Beds”, J. Chromatogr., 473, 273–275 (1989).
- T. B. Tennikova, B. G. Belenkii, and F. Svec, “High-performance Membrane Chromatography – A Novel Method of Protein Separation”, J. Liq. Chromatogr., 13, 63–70 (1990).
- F. Svec and J. M. J. Frechet, “Continuous Rods of Macroporous polymer as High-performance Liquid Chromatography Separation Media”, Anal. Chem., 64, 820–822 (1992).
- H. Minakuchi et al., “Octadecylsilylated Porous Silica Rods as Separation Media for Reversed-Phase Liquid Chromatography”, Anal. Chem., 68, 3498–3501 (1996).
- G. Guiochon, “Monolithic Columns in High-performance Liquid Chromatography”, J. Chromatogr. A, 1168, 101–168 (2007).
Emily Hilder is Professor and ARC Future Fellow in the Australian Centre for Research on Separation Science (ACROSS) and School of Chemistry at the University of Tasmania. Her research focuses on the design and application of new polymeric materials, in particular polymer monoliths, in all areas of separation science. She is also interested in the development of miniaturised analytical systems, particularly for applications in clinical diagnostics and remote monitoring. She has over 95 peer-reviewed publications and was recently recognised as the LCGC Emerging Leader in Chromatography (2012). She is also an Editor of the Journal of Separation Science.
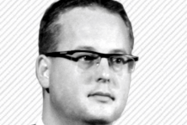
Sebastiaan Eeltink received his PhD degree in chemistry (specializing in analytical chemistry) in 2005 from the University of Amsterdam. Thereafter, he conducted postdoctoral research at the University of California, Berkeley, USA, and was guest scientist at the Lawrence Berkeley National Laboratory. In 2007, he joined Dionex and conducted research on packed and monolith column technology for ultra-high-pressure LC, twodimensional LC, and nanoLC. Eeltink is now research professor at the Department of Chemical Engineering at the Free University of Brussels, where he focuses on the development, characterization, and application of novel chromatography materials, including nano-structured monolithic materials and coatings in capillaries and micro-fluidic devices, for ultra-high-pressure and multidimensional (spatial) LC-MS separations.
Frantisek Svec lives in California and is Professor at the Beijing Advanced Innovation Center for Soft Matter Science and Engineering, Beijing University of Chemical Technology, Beijing, China and at the Department of Analytical Chemistry, Faculty of Pharmacy, Charles University, Hradec Králové, Czech Republic. He received a BSc in chemistry and PhD in polymer chemistry from the Institute of Chemical Technology, Prague (Czech Republic). In 1976 he joined the Institute of Macromolecular Chemistry of the Czechoslovak Academy of Sciences, before joining the faculty at Cornell University in 1992. In 1997, he was appointed at the University of California, Berkeley and also affiliated with the Molecular Foundry of the Lawrence Berkeley National Laboratory. Svec has authored 450 scientific publications, edited two books, and authored 75 patents. He is editor-in-chief of the Journal of Separation Science, member of editorial boards of a number of renowned journals and was President of CASSS in 2003–2015. He is best known for his research in the area of monoliths and their use in liquid chromatography, electrochromatography, supports for solid phase chemistry, enzyme immobilization, and microfluidics.
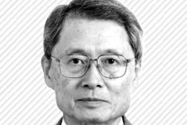
Nobuo Tanaka was educated at Kyoto University in Japan. After graduation, he spent several years as a postdoctoral researcher at University of Pennsylvania, University of Washington in Seattle, and finally Northeastern University in Boston. He returned to Japan in 1979 and worked his way up to full professor at Kyoto Institute of Technology where he was active for 30 years until his retirement in 2009. Since then, he has been a technical advisor at GL Sciences. Tanaka’s most important contribution was the development and reduction to practice of monolithic silica columns for HPLC. Now, he contributes to the development of high-speed, highefficiency monolithic silica columns and the operation methods that can enhance the performance of HPLC.