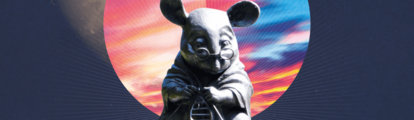
Of Mice and Monkeys
Animal models remain an unfortunate necessity in some fields of research and development, but analytical advances and new technologies are paving the way to reduce – maybe even one day eliminate – our reliance on living creatures
It’s not “antiscience” to highlight problems with animal models. Our (sometimes) furry friends have made many sacrifices on our behalf. In fact, estimates suggest that 110 million mice and rats are killed in US labs each year. And then there are the frogs… And dogs… And monkeys… And please spare a thought for the ubiquitous zebrafish…
But the use of animals in the pursuit of (scientific) knowledge is not only a modern-day practice. In ancient Greece, the dissection of human bodies was considered taboo and anatomical exploration thus relied on live animals. It was through conducting studies of this kind that Herophilus (“the father of modern anatomy”) started his own studies; the likes of Aristotle and Diocles also partook.
Today’s animal studies represent a significant departure from these barbaric practices. Subject suffering is limited wherever possible – and backed by legislation – and animals can even be housed with friends. But the drawbacks with animal models don’t start and end with ethical dilemmas – there are also concerns regarding their applicability to human disease and the logistics surrounding their maintenance.
Luckily, advances in analytical science are helping us to move forward in new directions. Advances in instrumentation and methodology are allowing us to use smaller samples (and thus fewer animals!), and new models that don’t rely on animals at all are emerging as alternatives.
Assuming that animal lovers outnumber the animal ambivalent, we decided an exploration of this exciting analytical frontier was long overdue.
Reduce, replace, refine
The three Rs initiative (replacement, reduction and refinement) for animal research began many years ago, providing widely accepted principles that guide the way we conduct animal studies. They ensure that we approach this work in the most efficient way possible when it is needed. An example: if these studies must be used, use the minimum number of animals possible. Another: extract the maximum amount of information from those animals. Cutting-edge analytical chemistry is crucial in achieving such feats.
“When I started in the pharma industry some 40 years ago (not in the 19th century, as some of my students may have thought), you needed over 40 rats to gather pharmacokinetic (PK) data, and a further six rats per dose route for the drug metabolism studies,” says Ian Wilson, a Visiting Professor at Imperial College, London. “This is because you needed 1 ml of plasma per time point for the LC-UV methods used in the PK studies, and this means you needed 2 ml of blood per sample. Six rats per time point were sacrificed to provide data of the required statistical quality. And that equates to an awful lot of rats.”
“Today, we can do the same study in three rats or mice,” he continues. “With modern analytical chemistry, we can assess PK and drug metabolism, and even conduct omics analyses using blood samples as small as fifty microliters in runs as short as five minutes.” In such cases, the combination of microsampling with chromatography and high-resolution MS can dramatically reduce animal usage while increasing data quality and hitting two of the three Rs: reduce and refine. Ian’s own work on gefitinib in mice, which used rapid UPLC methods followed by MS detection (1), is testament to these capabilities.
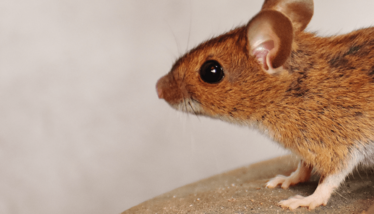
In tandem, the animal models that we use are also improving. It’s widely recognized that typical model systems like rat xenografts (in which human tumors are implanted into rats, treated, and monitored) have inherent limitations, such as their inability to accurately replicate the tumor microenvironment present in human patients. Newer systems, such as humanized livers (produced by infusing human hepatocytes into immunocompromised mice) or optically clear zebrafish xenografts (2), may be considered more “human relevant.”
From another perspective, analytical systems are also helping us identify and eliminate animal tests that are ineffective. As an example, researchers from AstraZeneca used 1H NMR spectroscopy to show that cynomolgus monkeys are poor models of paracetamol toxicity by studying their urine (3). Plasma exposure was higher than in humans, but paracetamol metabolites in the urine were rare. The conclusion: these monkeys do not metabolize the drug the same way humans do. And the outcome? Nobody should dose primates with paracetamol again!
The Zoo in Your Lab
A quick tour of the lab zoo – a collection of animals used for different types of testing around the world – with rapidfire reasons for their utility
Mouse (Mus musculus)
- Small size and large numbers of offspring
- Easy and cheap to keep
- Share many disease genes with humans
- Longstanding breeding programs mean that many different mice are available for different research purposes, such as immunodeficient and gene knockout mice
Zebrafish (Danio rerio)
- Easily kept due to low cost and small size
- Transparent eggs are fertilized and develop very quickly outside of the mother - ideal for developmental research
- Easy to manipulate genetically
African clawed frog (Xenopus laevis)
- Live up to 30 years in captivity
- Lay up to 1,000 eggs at a time - these eggs are relatively large and develop externally in salt solution, making them ideal for developmental research
- Take up little space and are easily bred and maintained
- Are the best understood amphibians
Dog (Canis lupis familiaris)
- Considered the most appropriate model for toxicology studies after rats
- Long history of use
- Many species are used, but beagles are the most common
Cynomolgus macaque (Macaca fascicularis)
- Offer unique insights into human biology and physiology due to evolutionary lineage
- Particularly useful for neurological and reproductive research
- Reproductive and developmental toxicology is well established
Nematode worm (Caenorhabditis elegans)
- Easily grown on plates of bacteria, and can be frozen, thawed, and revived as needed
- Has a short life cycle that lends itself to developmental studies
- Is transparent throughout its life, meaning its anatomy and individual cells can be studied with ease
- Specific development means that each cell can be traced back to the embryo stage
Organoids from outer space
In proving old models redundant or unfavorable, we not only spare animals the bother of ineffective studies, but we also underscore the need for alternatives. These alternatives can come in many shapes and sizes, but in vitro systems are one of the leading choices. But, as Ian says: “There’s no such thing as an in vitro rat just yet.” Luckily, we have organoids to take their place.
Organoids are three-dimensional organ models derived from stem cells that are able to mimic some of the complexities of tissues in the human body in terms of their spatial organization and cellular distribution. They represent a welcome departure from classical cell line and animal systems, which are limited by their culture-altered biochemical processes and physiological separation from humans, respectively.
Today, organoid models have been reported for almost every major human organ (including complex systems like the blood-brain barrier and fallopian tubes) and many of our most troubling diseases. Cancer is perhaps the most successful example. Oncological research has benefited from an organoid invasion due to their ability to replicate the pathophysiological features of naturally occurring tumor processes, such as growth and metastasis.
The application of high-throughput and omics profiling technologies, as well as MALDI imaging, allows us to evaluate drug effects in these in vitro tumors. And, in the case that organoids are patient-derived, these approaches can even help us to personalize treatment and improve outcomes for patients - both in cancer and beyond. The close interaction between organoids and analytical technology thus helps us to protect humans and animals alike, while also opening doors to innovative research approaches for the future.
On the topic of three-dimensional cultures, so-called “tissue papers” are also making a name for themselves. These systems use plant tissue material loaded onto paper-based scaffolds to mimic human tissues, allowing them to act as models for processes like cellular tissue invasion (common in cancer) (4). Tissue papers can also be implanted into animals with relative ease when the occasion calls for it as the plant-derived materials do not tend to elicit inflammatory responses!
Do you want chips with that?
“The advent of organ-on-a-chip technologies show great promise,” explains Steven Ray Wilson, a Professor from the Department of Chemistry at the University of Oslo. “These systems can be very representative of human functionality and they can be automated with high throughput and precision. They certainly represent a very attractive alternative to animals, but I don’t think that we can completely replace them just yet.”
The key tricky question that we must consider here is: can organ-on-a-chip technologies represent humans more accurately than animals? It’s a tricky question, but analytical science will likely lead the way to an answer.
“We must explore how these systems metabolize drugs,” Steven says. “And we must also map the protein makeup of these tiny and complex organ models if we are to compare them to the real counterparts in humans and animals. Some spectacular new breakthroughs suggest that they are very accurate indeed, as is the case with the embryo-like gastruloids. The field is exploding right now. Who knows where we will be in a couple of years!”
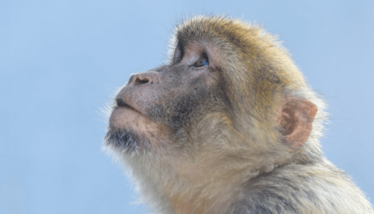
Of course, one core consideration in mimicking the human body is connectivity between organs; after all, when in practice does a human organ act totally alone? By connecting multiple organs on a single chip, researchers can study the interactions between the pancreas and liver in diabetes patients or the lung and brain in cancer patients. Analytical chemistry plays a central role here, too – often by the coupling of these systems to high-throughput MS systems for rapid readout.
As you might imagine, these inter-organ interactions are somewhat complicated and can differ greatly between species, so chip-based devices are a welcome addition to our research arsenal. We can even go one step further with “human-on-a-chip” technologies, which comprise many organs to replicate – to some degree – the whole human body. But if using animals for research is ethically questionable, how do we feel about using a “human-on-a-chip?”
Beyond the science
Such a question might seem odd or even silly at first. And my initial reaction to ethical considerations surrounding organs- and humans-on-chips was completely dismissive, but the field does raise unique and interesting concerns. A couple of quick questions from Ian highlight an area of potential concern: “At what point does a collection of glial cells constitute a human brain? How many interacting cells do we need to label something living?”
The next big question: if we consider these systems as living, how should we treat them? “It’s an interesting question,” admits Steven. “Thinking about it quickly leads us towards debates about the nature of life itself, and that provides an important opportunity for us to have interplay with the humanities. I imagine we will see more of this.”
Steven suggests that we should expect to see organ-on-a-chip technologies find a routine role in drug testing and developmental biology. In this latter application, ethical concerns become even more complex. For example, it is possible to mimic embryonic development by loading a chip with a spatially controlled patterning of pluripotent stem cells (4), but does that mean that we should?
Zebrafish are used widely for developmental studies because their eggs are fertilized and develop outside of the mother’s body. Observation is simple, and the fish are also cheap to house on a large scale. But how do we make the most ethical choice between experimenting with zebrafish versus embryo-like structures on chips?
From a research standpoint, it’s likely that both approaches will be employed to provide the most holistic view possible. And that is very much the case with animal models now; alternatives are here, but are often used in parallel with the minimum possible number of animal studies. Though this is likely to remain the case for some time, it’s exciting to imagine the doors that analytical technologies may open for us (and our animal friends) in the years to come.
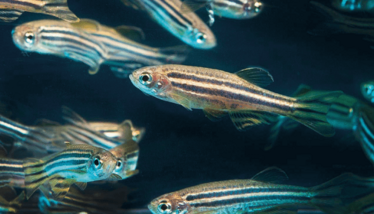
The ubiquitous zebrafish
- BJ Malloy et al., “Rapid determination of the pharmacokinetics and metabolic fate of gefitinib in the mouse using a combination of UPLC/MS/MS, UPLC/QToF/MS, and ion mobility (IM)-enabled UPLC/QToF/MS,” Xenobiotica, 51, 434 (2021). DOI: 10.1080/00498254.2020.1859643
- C Yan et al., “Visualizing engrafted human cancer and therapy responses in immunodeficient zebrafish,” Cell, 177, 1903 (2019). DOI: 10.1016/j.cell.2019.04.004
- H Yu et al., “Metabolism by conjugation appears to confer resistance to paracetamol (acetaminophen) hepatotoxicity in the cynomolgus monkey,” Xenobiotica, 45, 270 (2014). DOI: 10.3109/00498254.2014.973000
- SM Cramer et al., “Tissue papers: leveraging paper-based microfluidics for the next generation of 3D tissue models,” Anal Chem, 91, 10916 (2019). DOI: 10.1021/acs.analchem.9b02102
- A Manfrin et al., “Engineering signaling centers for the spatially controlled patterning of human pluripotent stem cells,” 16, 640 (2019). DOI: 10.1038/s41592-019-0455-2
I've always wanted a job that fosters creativity - even when I worked on the assembly line in a fish factory. Outside work, I satisfy this need by writing questionable fiction. The venture into science writing was an unexpected departure from this fiction, but I'm truly grateful for the opportunity to combine my creative side with my scientific mind as Editor of The Analytical Scientist.