Pharma's DBS Dilemma
Advantages of dried blood spot (DBS) analysis for the pharmaceutical industry abound yet analytical and regulatory challenges are hindering widespread uptake. Here, two leading proponents of the approach discuss what can be done to overcome the barriers to its implementation.
Neil Spooner, Paul Abu-Rabie |
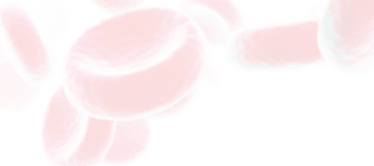
There has been much recent interest in the use of dried blood spot (DBS) sampling for the determination of circulating drug concentrations, particularly in support of clinical and non-clinical studies performed as part of regulated pharmaceutical development (1, 2).
DBS offers an alternative to conventional wet plasma sampling, which is widely employed in pharmaceutical development due to ease of handling, shipping and storage compared to whole blood, and its historical acceptance by practitioners and regulators.
DBS’s primary advantage over conventional plasma sampling is that it is readily employed as a microsampling technique. In practice, DBS sampling – typically involving the collection of 3 x 15µl spots – requires 50 – 100 µl of blood, including unavoidable wastage, compared to ~500 µl for conventional plasma sampling (2). This volume reduction provides an ethical advantage, particularly for toxicology studies with rodents. The physiological effects of conventional blood sampling approaches mean that extra study animals are added (known as toxicokinetic (TK) satellites) to obtain good quality drug exposure data, without compromising other endpoints for the main toxicology study animals. With DBS, TK samples can be taken from central study animals, reducing the overall number of animals needed. Using DBS as a microsampling approach is an important step forward in demonstrating a progressive approach to animal use. Furthermore, warming of rodents is required to obtain sufficient blood volumes for conventional sampling, which can be stressful to the animals. Overall, DBS is a significant contribution to the implementation of the 3Rs (replacement, reduction and refinement) in animal use for drug development.
A further benefit of microsampling is that the reduced sample volumes enable clinical pediatric studies to be performed, particularly in very small children, and facilitate the juvenile toxicology studies that are required to support pediatric registration. These studies, which would otherwise be difficult or impossible to conduct, support the provision of medicines for children and potential patent life extensions.
Other DBS benefits include reduced shipping and storage costs, simplified procedures, and a reduction in the amount of test substance required. Samples for many analytes can be shipped and stored at ambient temperature, eliminating the need for refrigeration or freezing. This generates savings that are particularly notable for multi-site clinical studies; shipping at ambient, rather than frozen temperatures, can reduce costs by tens or hundreds of thousands of Euros (3).
Chemically treated DBS paper substrates lyse cells and denature proteins on contact, meaning that sample shipping can sometimes be carried out without the extra costs incurred when handling potentially harmful biological samples [web reference: a]. DBS also offers the potential of less invasive sampling – via a finger or heel prick, rather than venous cannula in human studies – especially when the blood sample is only for pharmacokinetic assessment. This may aid recruitment of subjects for clinical studies, which is a perpetual problem for drug development projects.
The fact that DBS sampling eliminates the need for centrifugation of blood and freezing of the derived sample makes it ideal for Phase II/III drug development studies in locations where specialist equipment and consumables for sample processing are not readily available. Similarly, it is ideally suited to drug screening where the collection of wet samples is not feasible. Another potential application is therapeutic drug monitoring to enable sampling in a patient’s home or local doctors surgery. Finally, there are also advantages for monitoring critically ill patients, where it may be important to minimize the volume of blood being taken.
So, with all the advantages DBS has on offer, why hasn’t it already replaced conventional wet plasma analysis as the primary sampling technique to support pharmaceutical development? The remainder of this article explains the barriers to the widespread adoption of DBS sampling for regulated drug development, and the prospects of the technique.
Bioanalytical barriers and direct analysis
Current interest in DBS for regulated quantitative bioanalysis stems from work initiated by bioanalytical groups. Somewhat ironically, one of the major barriers to the broad acceptance of DBS sampling also comes from bioanalytical scientists.
Compared to conventional wet plasma analysis, the DBS extraction procedure is more complicated, assay sensitivity is lower (microsampling means less sample, and thus, less drug is available to be analysed), and ion suppression is higher. There is understandable reluctance to switch from a tried and tested technique that is accepted by regulatory authorities to one that is more complex and that is under greater regulatory scrutiny. Given the overall benefits to patients and drug development organisations, these challenges should perhaps be a minor inconvenience but any barrier could dilute the ethical and financial advantages.
You could be forgiven for thinking that the need to punch out a disc from a DBS sample is a very minor increase in extraction complexity and, in isolation, this is certainly true. Indeed, it barely involves any more effort than the equivalent step in conventional wet plasma analysis, which is aliquotting an accurate volume into a sample tube using a pipette. However, considered from a high-sample-throughput perspective, where a staff member will analyse hundreds of samples per day, this can become a rate-limiting step, severely impacting efficiency. In conventional (high throughput) wet plasma analysis, this problem has been eliminated by introducing the use of automated liquid handling robots, which reduced this part of the process from hours to minutes and allowed the analyst to undertake other activities whilst their samples were being processed. This partly explains the consternation of some bioanalysts faced with going back to a manual process of individually punching DBS samples. To curtail this issue, the manual handling limiting factor also needs to be addressed for DBS sample extraction. For many bioanalysts to even consider DBS to be a suitable alternative, the effort involved with sample extraction needs to be on a par with conventional wet plasma analysis.
Automated DBS analysis
Semi- or fully-automated ‘card-punching’ instruments [b, c, d] can be used in tandem with a liquid handling robot [e] to relieve some of the manual burden of DBS analysis. Such instruments are available that use the workflow bioanalysts are accustomed to, which is a clear advantage, but they do not deliver the seamless workflow provided by direct elution and direct desorption. The numerous automated DBS analysis instruments used in our laboratories have not been overwhelmingly popular with our analysts.
Direct analysis
Direct analysis encompasses direct elution and direct desorption techniques, and describes any technique that eliminates the manual steps in sample extraction, including punching the DBS. The ideal workflow is simple: DBS samples are loaded onto the direct analysis instrument and extraction, separation and detection of the analyte is completely automated (see Figure 1).
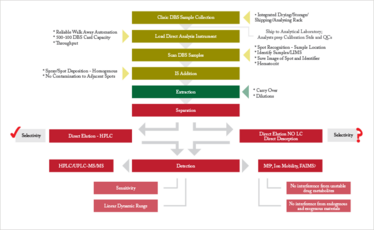
Figure 1: DBS direct analysis theoretical workflow
In direct elution (also known as direct extraction), the analyte of interest is extracted with a liquid solvent, without sub-punching the spot. Specific techniques include on-line DBS; liquid microjunction-surface sampling probe (LMJ-SSP) and liquid extraction surface analysis (LESA) [f]; the sealing surface sampling probe (SSSP); and digital microfluidics (DMF) (4–8). The mechanism of extraction is conventional liquid extraction but it uses a sealed sampling area or confined liquid stream, low extraction solvent volumes, and direct introduction of the extract into the detector. These techniques are very well suited to DBS direct analysis. Crucially, unlike most direct desorption techniques, direct elution produces a liquid extract that is compatible with the currently used HPLC-triple quadrupole MS/MS bioanalytical workflow, which is an important regulatory and financial consideration. In addition, DBS direct elution offers an order of magnitude increase in assay sensitivity compared to manual extraction, as the entire area of the spot eluted is directed onto the HPLC column for analysis, rather than a subaliquot being injected for sub-punching and elution workflows (9). This is an important factor since low sensitivity limits the application of DBS in the pg/ml region, for example in studies of low dose or inhaled drugs.
A major concern was that the elimination of sample clean-up in direct elution techniques would results in a ‘dirtier’ extract being introduced into the MS, potentially compromising the robustness of the analytical procedure. However, to date, no studies have found a significant difference in robustness between DBS direct elution and manual extraction (10).
Development of the functionality of direct elution instruments has made them compatible with the workflow detailed in Figure 1 [g, h, i], including:
- Sample capacity of up to 500 DBS cards
- Fully automated card handling
- Intelligent visual recognition systems to recognise the quality of samples and where to elute
- Integral wash stations to reduce cross contamination
- Integrated internal standard (IS) modules that allow the analyte and IS to be co-extracted (11).
The emergence of commercially available, fully automated instruments make the use of DBS in regulated quantitative bioanalysis a realistic prospect in the near future.
Direct desorption
Here, we use the term ‘direct desorption’ to describe direct analysis techniques that use non-liquid elution for analyte extraction and do not produce a liquid extract that is compatible with HPLC. This encompasses techniques that have also been referred to as atmospheric pressure surface sampling/ionisation MS; ambient ionization MS; ambient MS, and ambient desorption ionization MS techniques.
These techniques include thermal desorption, for example, direct analysis in real time (DART); laser desorption, for example, laser ablation electrospray ionization (LAESI); and gas jet desorption, for example, desorption electrospray ionization (DESI).
One of the potential advantages of direct desorption, namely the elimination of liquid chromatographic (LC) separation, is also one of the challenges for successful introduction into a regulated bioanalytical environment. While eliminating LC would simplify the bioanalytical workflow, offering greater sample throughput, its removal may result in poor sensitivity due to ion suppression, reduce selectivity, and risks assay interference via metabolite decomposition into parent compounds during MS ionization.
It is possible that different types of separation techniques than those commonly found in the bioanalytical laboratory (ion mobility, FAIMS, or some novel approach) will overcome these drawbacks (see Figure 1). Significant resources, both financial and time, are required to develop and underpin these techniques to ensure that they are acceptable for regulatory approval.
This is not the only barrier to the practical application of direct desorption. A direct desorption technique that offers adequate sensitivity across a range of representative pharmaceutical small molecule compounds has yet to be described. DBS analysis using DART on the widely used cellulose paper substrate format is considerably less sensitive than manual DBS extraction. DESI (12), and in particular Paper Spray (13), has sensitivity close to, and in some cases exceeding that of manual DBS extraction for some compounds, but is disappointing for others.
In the regulated quantitative environment, direct desorption is a long-term goal. In the meantime, it has found a useful role in applications such as drug screening and therapeutic drug monitoring.
The hematocrit issue
Hematocrit (HCT) values that deviate from the ‘normal’ expected range can significantly affect assay bias when using a sub-punch approach to obtain the sample for the quantitative bioanalysis of drugs (14). The issue can be broken down into three components: area bias, recovery bias, and ion suppression bias (15).
The area bias is the influence of the viscosity of the whole blood sample, which is directly related to HCT. For a fixed volume of blood on cellulose substrate, high HCT produces a relatively small spot area; low HCT a relatively large area. Consequently, the analyte density of each spot is different because the area that a fixed volume of blood will spread over depends on the HCT value and a sub-punch taken within the sample will contain differing amounts of matrix. The recovery of analyte can also be dependent on the degree of hematocrit. The effect can be the opposite to that of the blood spot area, causing an apparent reduced overall effect on analytical data. Clearly, if DBS sampling is to be used for regulated quantitative bioanalysis to support pharmaceutical development, the relationship between DBS sample HCT and assay bias is a key issue.
The ideal solution for controlling the area bias would a novel substrate that behaves independently of HCT, which would enable the current workflow to remain unchanged. It could be a novel substrate material, substrate configuration or the addition of a modifying agent that, for example, eliminated differences in drying rates. Such a modifier could be added to the sample prior to spotting although this would compromise the simple workflow. Unfortunately, such a substrate or modifier has yet to be reported. Currently, the effect of HCT on assay bias is assessed during method validation to ensure that bias is within acceptable limits, which are typically <±15% (16). However, HCT values for study samples may be unknown and certain disease states, or exposure to certain drugs, could take a patient’s hematocrit level beyond normal ranges.
The most practical way to avoid HCT based DBS area variability at the moment is to extract the whole spot rather than take a sub punch. However, to ensure confidence in the analytical result, an accurate volume must be dispensed at the clinic – a general inability to do so is the reason for taking a sub punch in the first place! Reliably dispensing accurate volumes in a clinical setting is a challenge. Blood collection devices must be developed that are accurate, cheap, disposable, and faster and easier to use (by non-analysts) than conventional positive displacement pipettes.
Existing automated DBS analysis and direct elution techniques can be made compatible with whole spot elution through relatively minor modifications, such as larger punches or sealed sampling areas. Another advantage of whole spot extraction/elution is that sample volumes could be further reduced without affecting assay sensitivity, as the same amount of blood could be sampled in total as is currently sampled in the sub punch, eliminating the wastage currently associated with manual extraction. Alternatively, current DBS volumes could be retained, eliminating many of DBS’s sensitivity issues. It is not currently clear how hematocrit level effects direct desorption techniques, but it can be assumed that the area bias will be relevant in any analysis technique that samples a localised area rather than the whole sample. Further investigation is required into the mechanisms of direct desorption sampling and how hematocrit variation will bias analytical results.
High HCT levels often result in lower analyte recovery, an issue that becomes increasingly significant as overall assay analyte recovery decreases (17). Maximising the recovery of analytes will reduce the range of the recovery bias as HCT changes. An improved method of IS addition, that allows the analyte and IS to be co-extracted, would greatly assist in this regard as would additional sample clean-up to remove interfering species and/or modifying chromatography to move the analyte away from areas of suppression. The hematocrit issue certainly complicates the ultra-simple workflow on offer from DBS sampling, but the introduction of an elegant sampling device in the clinic, coupled to a fully automated direct elution instrument, should enable the benefits of DBS to be reaped without major compromise.
Regulatory issues
Whole blood is a suitable matrix for pharmaceutical drug development studies (18). However, as with any new technique in this highly regulated field, DBS faces intense scrutiny from regulatory authorities, whose role it is to ensure maximum confidence in the data produced in support of new drugs, and ultimately that the drugs are safe for public consumption. A considerable body of research is required to prove any new technique. Currently, guidance from the US Food and Drug Administration (FDA) requires the collection of DBS and wet matrix data in parallel for a subset of samples for any particular drug development program, to prove concordance between the two sample methods (19). If this is clearly demonstrated, then it is likely that the regulator will allow DBS sampling to be used on its own for subsequent studies. Presumably, once enough positive data has been collected for a number of molecules, regulators will deem DBS to be reliable and relax the requirements. In the short-term, however, the introduction of DBS faces a financial hurdle. Supporting parallel sampling and analysis greatly increases costs, which project managers and drug development organisations are reluctant or unable to bear. Given that so many are unwilling to make the required financial commitment, progress in DBS has slowed after the initial surge of interest.
We hope that the clear long-term benefits will drive the establishment of DBS. A number of organisations are already using DBS sampling for studies where this approach can demonstrably provide benefits over conventional wet sample – good examples include clinical trials in remote areas, studies in small children and pre-clinical rodent microsampling. Publication of these data will enhance understanding of the technique. In addition, consumable manufacturers and vendors see the potential benefits of DBS and are working to overcome, or minimise, the issues associated with HCT and homogeneity. When these projects come to fruition, DBS sampling will rival wet plasma sampling in regulated drug development. To help accelerate the process, a number of industry consortiums, such as the European Bioanalysis Forum (EBF) and the International Consortium for Innovation and Quality in Pharmaceutical Development (IQ), are running projects and sharing the data with the wider community. These multi-company initiatives should generate broader acceptance of the technique for use in regulatory quantitative bioanalysis as part of drug development.
Paul Abu-Rabie and Neil Spooner work in Platform Technology and Science, Drug Metabolism and Pharmacokinetics, at GlaxoSmithKline Research and Development, in Ware, UK. Abu-Rabie is also affiliated with the School of Science, University of Greenwich in Kent, UK.
Web References
a. www.Whatman.com
b. www.bsdrobotics.com
c. www.perkinelmer.com
d. www.hudsonrobotics.com
e. www.hamiltonrobotics.com
f. www.advion.com
- M. Barfield et al., Journal of Chromatography B 870 (1), 32-37 (2008)
- N. Spooner, R. Lad, M. Barfield, Analytical Chemistry 81 (4), 1557-1563 (2009).
- N. Spooner, Bioanalysis 5 (8), 879-883 (2013)
- J. Déglon et al., Journal of Pharmaceutical and Biomedical Analysis 49 (4), 1034-1039 (2009).
- G. J. Van Berkel, V. Kertesz, R. C. King, Analytical Chemistry 81 (16), 7096-7101 (2009).
- V. Kertesz and G. J. Van Berkel, Journal of Mass Spectrometry 45 (3), 252-260 (2009).
- H. Luftmann, Analytical and Bioanalytical Chemistry 378 (4), 964-968 (2004).
- M. J. Jebrail et al., Lab on a Chip (19), 3218-3224 (2011).
- P. Abu-Rabie and N. Spooner, Analytical Chemistry 81 (24), 10275-10284
- (2009).
- P. Abu-Rabie and N. Spooner, Bioanalysis 3 (24), 2769-2781 (2011).
- P. Abu-Rabie et al., Analytical Chemistry 83 (22), 8779-8786 (2011).
- J. M. Wiseman et al., Analyst 135 (4), 720-725 (2010).
- N. E. Manicke et al., Journal of the American Society for Mass Spectrometry 22 (9), 1501-1507 (2011).
- P. Denniff and N. Spooner, Bioanalysis 2 (8), 1385-1395 (2010).
- P. Denniff and N. Spooner, “Effect of Hematocrit on Quantitative Dried Blood Spots”, presentation at The 19th International Reid Bioanalytical Forum, 2011.
- V. P. Shah et al., Pharmaceutical Research 17 (12), 1551-1557 (2000).
- P. Denniff et al., “Issues with DBS and HCT - Possible Solutions”, presentation at the British Mass Spectrometry Society Annual Meeting, 2012.
- ICH Guideline, “Guidance on nonclinical safety studies for the conduct of human clinical trials and marketing authorization for pharmaceuticals M3 (R2)”, 2009.
- Y. Xu et al., Bioanalysis 5(3), 341-350 (2013).
Neil Spooner is founder and director of Spooner Bioanalytical Solutions and the Senior Editor of Bioanalysis, the journal for the field of regulated quantitative bioanalysis. He is also senior visiting research fellow at the School of Life and Medical Sciences, University of Hertfordshire, UK.
Paul Abu-Rabie has been part of the Drug Metabolism and Pharmacokinetics (DMPK) group at GlaxoSmithKline, Ware, UK, for the last 10 years. He is currently a technical leader in the Bioanalytical Science and Toxicokinetics (BST) department supporting small molecule and biopharm applications. His main areas of interest include the development of new technology and innovations in bioanalysis, particularly in mass spectrometry, microsampling and direct analysis. Paul received a degree in Chemistry in 1998 from the University of Sussex, UK. He is currently undertaking a PhD on the topic of direct analysis under the tutorship of Professor Frank Pullen at Greenwich University, UK.