Ten Year Views: With George Chan
George Chan discusses the decade’s developments in analytical atomic spectrometry, concerns for the field, and future predictions
Georgia Hulme | | 6 min read | Interview
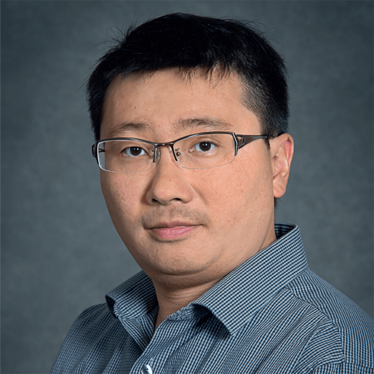
Could you give us a brief introduction to analytical atomic spectrometry? What falls within its scope?
It is logical to refer to analytical atomic spectrometry as analysis for elemental (atomic) information based on the principle of either electromagnetic radiation (optical spectrometry), or charged particles (mass spectrometry).
On the optical spectrometry side, techniques are typically classified according to their working principles – absorption, emission and fluorescence – with a wide variety of atom reservoirs and excitation sources. The wavelength range is vast, spanning from x-ray to near-infrared, although only a subset is used in almost all cases.
On the mass spectrometry side, there is also a wide range of ionization sources coupled to different types of mass spectrometers, and often, the same atom reservoir can be used as an excitation source in optical, and mass spectrometry. There are also some “hybrid” techniques that use optical transitions to achieve selective ionization – followed by detection of the charged particles. In addition, sample introduction techniques, instrument hardware, data handling, processing and reduction techniques – specific for use with the spectrometric methods – all fall within the scope of analytical atomic spectrometry.
What has been the most significant development in atomic spectrometry over the past 10 years?
There have been several significant developments. I think that the ChemCam instrument – which was installed on the Mars Curiosity Rover and landed on Mars in 2012 – is the most significant. ChemCam combines a high-resolution remote imaging camera and a laser induced breakdown spectroscopy (LIBS) system to characterize the elemental composition of rock and soil samples on Mars. Although the development of LIBS is not new (it can be traced back to the 1960s shortly after the laser was invented), LIBS was still more like a laboratory research tool at the start of the 21st century. Sending a LIBS-based instrument to Mars shows the analytical community how advanced, capable, and reliable the LIBS technique and hardware is. The success of ChemCam, of course, is driven by the needs of a special mission, but advancement of the technology, and reliability of lasers and photodetectors are the keys to its success.
What have been the most important commercial developments over the past decade?
Array detectors are indispensable tools in optical spectrometric measurements – but they are not without their drawbacks. One limitation is that registered “detector counts” can not be readily reverted back to the number of photons or photoelectrons (for various reasons). Although some modern array detectors can be used in a photon-counting mode, the dynamic range is then greatly reduced as a pixel is temporarily “dead” after a single photon hits it – a phenomenon similar to detector dead-time in ion-counting mass spectrometry. An array detector with read noise significantly less than one electron only became available two years ago. In particular, the readout noise of the ORCA-Quest qCMOS camera from Hamamatsu is reported to be 0.27 electrons rms. This low level of read noise makes counting of multiple photoelectrons on a single detector-pixel possible. Although the global reset time of this camera is not yet fast enough to match the typical timescale of LIBS measurements, I consider it an important innovation in detector technology.
Do you have any concerns for the field?
My major concern involves the continuation and transfer of experience and knowledge to the next generation. There are several reasons for this concern. Firstly, without dispute, the academic community of analytical atomic spectrometry has been shrinking for the past 20 years. In almost all cases, retired faculty members specializing in analytical atomic spectrometry have been replaced by someone specializing in another area. As a result, there are now only a small number of active research groups that can transfer knowledge in atomic spectrometry to the next generation.
Secondly, the focus of many research programs has shifted from fundamental understanding and instrument development to method development and applications (for example, metallomics, speciation, forensics, environmental). A natural consequence of a heavy focus on applications is a de-emphasis on fundamental theory and mechanistic studies. Experience, knowledge, and expertise in the field of atomic spectrometry are often lost and not transferred to students or technicians.
Another concern is the potential harm that may arise from the ever-increasing computation power available for data mining, pattern recognition, and chemometrics. These are powerful tools, but there is also a danger of giving users a false impression that training in reading and understanding spectral data is old fashioned and no longer important.
A similar situation involving a lack of knowledge transfer to the next generation was noted about a decade ago in the field of nuclear science and engineering. To combat this problem, the US Department of Energy initiated several university consortia with generous support to ensure that a strong pipeline of new technical talent would be available in the future. I hope that someone with vision and power will see that a similar situation is developing in many other disciplines, including, but not limited to analytical atomic spectrometry, and take action before it’s too late.
What are you most excited about?
In short, the joy of working with good people is what excites me most about the spectroscopy field. I also like the intellectual challenge of solving difficult problems with my expertise. In particular, I like to conduct fundamental studies that explain new experimental observations and also explore novel applications with new tools. I am fortunate enough to continue conducting fundamental research even though my current position is not at a university. How? Well, some applications require fundamental characterization before methods can be formulated – one example is my project exploring the use of LIBS for optical isotopic analysis of uranium. We need fundamental details of the uranium LIBS plasma and an understanding of the behavior of the various uranium emission lines.
In some other situations, fundamental research is performed through collaborations with my friends at universities. For example, my collaborators and I submitted a manuscript in December 2022 on characterizing a plasma-based ambient desorption-ionization source using temporally resolved monochromatic imaging spectrometry. It was a fun project, and the idea of writing this work up for submission developed from a casual chat with Carsten Engelhard, University of Siegen, and Jacob Shelley, Rensselaer Polytechnic Institute, at a conference this past October. The data was not new, but we now have a different and better explanation compared with when we originally collected the results.
Overall, where do we go from here?
Development is highly funding-driven. I can see there will always be a need in analytical atomic spectroscopy for elemental and isotopic analysis. In addition, there will be a demand from users and stakeholders for techniques and instruments that, compared with current tools, are smaller, easier to use, while providing higher sensitivity and faster analysis times. The question is whether there will remain a sufficient supply of well-trained analytical spectroscopists for ongoing development.
DISCLAIMER: The views and opinions of the author expressed herein is of his own and do not state or reflect those of the United States Government or any agency thereof or the Regents of the University of California. The full disclaimer can be found online.
Georgia Hulme is Associate Editor at The Analytical Scientist