When HPLC was “discovered” over 50 years ago, it revolutionized the field of analytical chemistry. Major developments in the technique – which seemed to occur almost yearly in the 1970s and the early 1980s – ranged from revolutionary to evolutionary. During that period, the technique quickly shifted from a large-particle, gravity-fed, large-bore glass column technique to an automated, small-particle, high-pressure, narrow-bore stainless steel column technique. In the story of HPLC development, the column and the instrument are intertwined – and both have played pivotal roles in increasing separation speed, boosting efficiency, and improving quantitation.
In this article, we attempt to identify – with the help of a panel of LC, data systems and mass spectrometry experts (see “We Couldn’t Have Done It Without You”) – the developments that truly “made a difference.” We believe the resulting Top 10 allowed HPLC to surpass most other techniques in terms of application range and its ability to answer analytical questions. And so, without further ado...
The Top 10
Some of the chosen products in the Top 10, though not necessarily the best sellers or performers, were the first to be introduced into the market and being first gave other instrument developers a target to beat, driving further advancements in the technology ending with today’s UHPLC.
1. LCS-1000
Year: 1967
Company: Picker Nuclear
The first commercial integrated instrument to qualify as a modern LC instrument, including a UV 254nm detector, acquired by Varian Associates in 1968.
2. 6-Port Injection Valve
Year: 1968 Did away with septum injector and stop-flow techniques, allowed high-pressure injections, improved retention time, reproducibility, automation and quantitation. |
![]() | |
---|---|---|
3. Modular Components
Year: 1968 Components like the standalone 254 UV detector, LDC RI detector and Milton Roy minipump were used quickly by modular chromatographers. The first instruments were integrated, with all internal parts in a single box. The modular market sprung up to allow researchers to get the best component (for example, pump, injector, column holder/oven, detector). LDC was the first component company to step up and supply affordable and functional modules. |
![]() | |
---|---|---|
=4. Autolab System IV Computing Integrator
Year: 1969
Company: Autolab
The first automatic integrator for chromatography replaced cut and weigh, planimeters and recorders with built-in mechanical integration. This data system was first used in GC and adapted to LC. Features included tangent peak detection, baseline correction and normalized peak areas, and allowed the use of response factors and internal standards. Autolab acquired by SpectraPhysics in 1969.
=4. M-6000 pump
Year: 1972 First pump designed specifically for HPLC. Key features: dual reciprocating, less need for pulse damper, 6,000 psi output, and a low-volume chamber. |
![]() | |
---|---|---|
5. Model 708AL Autosampler
![]() | Year: 1974 The first automated sampling device for HPLC; improved throughput and quantitation ability. Future products improved on this unit. | |
---|---|---|
6. HP 1084
Year: 1976 Integrated HPLC system with automated sample injection, flow control, UV detector control, recording and reporting. First LC with digital processor control, with a builtin keyboard with push button control. It became the gold standard for quality and performance for many years. |
![]() | |
---|---|---|
7. 8450 Diode Array Detector
Year: 1977
Company: Hewlett-Packard
The diode array detector allowed on-the-fly UV-VIS spectra during the chromatographic process, with excellent signal-to-noise performance. Productivity increased compared with stop-flow spectral scanning. Other companies soon followed with their own diode arrays.
8. Moving Belt LC-MS Interface
Year: 1976
Company: Finnigan MAT
The moving belt was introduced by McFadden, Schwartz & Bradford of Finnigan MAT (6). Despite some drawbacks, the moving belt provided true chromatographic interfacing, the first successful LC-MS interface on the market, and was the best of many approaches – until electrospray came along.
9. Charged Aerosol Detector (CAD)
Year: 2005 Sometimes described as “the poor man’s mass spectrometer,” the CAD is a universal detector. It has much greater sensitivity than the RI detector (also a universal detector). It is still in widespread use today. |
![]() | |
---|---|---|
10. Acquity UPLC
Year: 2004 For the introduction of sub-two micron particles, new higher pressure instruments were required. The Waters Acquity UPLC system was the first system designed to meet the needs of the new small particle columns with a pressure output of 12,000 psi. Lower extra column effects and other embellishments were added to Acquity to meet the goals of modern UHPLC. |
![]() | |
Honorable Mentions
Choosing a Top 10 was not easy. To enrich the story (and to help us sleep better at night), we also decided to highlight those technologies that strongly influenced modern HPLC technology but didn’t quite make the cut.
1a. ALC-100
Year: 1967
Company: Waters Associates
The image above shows Robert Burns Woodward with his Waters ALC-100. This was a modified GPC-100 with lower dead volume. It was the first, most popular general-purpose instrument.
1b. 820
![]() | Year: 1969 Integrated with a constant pressure pump, Model 410 UV detector, oven and Dupont ZIPAX SPP columns. | |
---|---|---|
2a. U6K Injector
![]() | Year: 1973 Developed specifically for HPLC; loop by-pass to prevent column shock, 6000 psi capability, variable injection volume. | |
---|---|---|
2b. 7125 Valve
Year: 1976
Company: Rheodyne
Allowed syringe injection into the valve center and became greatly popular. Later used as OEM by many instrument companies.
3a. CE212
Year: 1971 Standalone first variable wavelength detector with stop-flow scanning. |
![]() | |
---|---|---|
4a. 4100/4200 Syringe Pump
Year: 1970 A new pumping principle and the first syringe pump for high-pressure operation and pulseless flow. Succeeded by the Model 8500. The isocratic (Model 4100) gave stable flow rate, while the gradients (Model 4200) suffered from solvent bulk compressibility, affecting flow and solvent composition reproducibility. Varian is now a part of Agilent Technologies. |
![]() | |
---|---|---|
4b. 110 Pump
Year: 1976
Company: Altex
First affordable standalone reciprocating pump with optimized duty cycle via variable piston speed, which lowered flow pulsations. The 110 gave a smoother flow, better baselines and improved quantitation, and set the tone for future pump development.
5a. HP 3380A Digital Integrator
![]() | Year: 1974 Microprocessor-controlled integrator with a chart recorder and alpha numeric printer-plotter. A ll calculated data given on one piece of paper. | |
---|---|---|
5b. PC Software
Year: 1979 Used PC as a driver for peak integration, quantitation and instrument control,. OEM to many manufacturers. After the Nelson SW, almost all instruments used a PC to control the hardware and to handle the data; standalone computing integrators died a rapid death (company acquired in 1989 by Perkin Elmer). |
![]() | |
---|---|---|
6a. LC 5000
Year: 1977
Company: Varian Associates
A completely new approach to an integrated system. The Pump was designed to accept three solvents into a single pump head with low-pressure mixing. The system had its own CRT and keyboard, detectors could attach side on, and aspects of LC except the data system - which was external - were handled by microprocessor. This system set the stage for most LCs to follow
6b. Model 10 Ion Chromatograph
Year: 1975 Durrum instruments acquired patents from Dow Chemical and started a separate division (Dionex) to develop a product to separate ionic compounds with conductivity detection using a suppressor column to remove salts from mobile phase and reduce background conductivity. Dionex became a leader in this technology and remains #1 today (now part of Thermo Scientific). |
![]() | |
---|---|---|
7a. Thermospray LC-MS Interface
Year: 1987 EI-type fragmentation sometimes obser ved as was signif icant fragmentation of protonated or deprotonated molecules. Low sensitivity was achieved for nonvolatile analytes across a limited range of LC conditions. This became the primary LC/MS interface until the 1990s, primarily due to the observation of EI-type fragmentation. |
![]() | |
---|---|---|
7b. Electrospray (Ion Spray) LC-MS Interface
Year: 1989 John Fenn (Yale University and independently) developed an electrospray ionization (ESI) source that produced intact, high-molecular-weight, multiply protonated or deprotonated ions. Fenn received the Nobel Prize in Chemistry in 1992 for this discovery. His acceptance speech was titled “When Elephants Fly.” ESI sensitivity was observed to be analyte-concentration-dependent. Peaks eluting from capillary columns are significantly sharper than from wider bore columns, leading to greatly increased sensitivity with lower injection volumes. Now, with various other API techniques, this is the standard MS interface for LC. Henion et al developed (1) ion spray interface that used nebulized nitrogen to assist electrospray operation; SCIEX used the Henion patent (2) and was first to market the product. |
![]() | |
---|---|---|
The Top 10 narrative
To simply rank the Top 10 does not do justice to their impact. Over the following pages, we tell the story behind the Top 10 (and the honorable mentions), giving much needed context – and a history lesson to all but the most seasoned LC users!
The mighty column
Column technology was one of the main drivers in instrumental development. Figure 1 shows a historical chart of commercial HPLC particle development. The work of Csaba Horváth and coworkers from Yale University in New Haven, CT (1) in 1967 on pellicular packings (now referred to as superficially porous packings, SPP) represented a breakthrough in HPLC column and instrument technology. Fifty-micrometer spherical glass beads were coated with a thin polymeric layer (1-3μm) of polystyrene resin, derivatized to form anion exchange functionality and used for the separation of nucleotides. The particles were packed into stainless-steel columns (1 mm ID, 3 m length), which gave rise to high back pressure and the need to use pumps to push solvents through the column. Notably, we see the beginning of a trend where smaller particles increase column efficiency (plates) to provide better separation performance, while also increasing column back pressure with the inverse square of the average particle diameter; thus, pump output pressure would become an important factor over the following years. But it was not the only driver; as we will see, parameters like flow rate, extra column effects (system dead volume), decreased peak widths, speed of elution, sample throughput and detector capability all became drivers to improve all parts of the LC system.
The first HPLC generation
In an earlier publication (2), a case was made that the work of Horvath and colleagues breakthrough work was the beginning of HPLC (at the time referring to high pressure LC). A schematic of their gradient elution instrument is depicted in Figure 2. Their home-made system used two Milton Roy Minipumps (Riviera Beach, Florida). The gradient was formed by starting with a stirred reservoir containing the weak solvent, and then having a pump deliver a controlled but increasing amount of the strong solvent to the weak solvent reservoir. A second high-pressure pump pushed the solvent mixture of increasing strength from the reservoir to the column. For detection, a modified spectrophotometer with an 8-μL flow cell was used, indicative of future developments. In the late 1960s, they teamed up with a local instrument company, Picker Nuclear (White Plains, NY), to construct the LCS-1000 – the first true commercial HPLC instrument. The design was spearheaded by engineer Emmett Watson, who had left Waters Associates to become a consultant. The final instrument had a high-pressure pump (up to 4000 psi), a sampling loop valve, an oven for temperature control, the column with the pellicular packing mentioned above, and a fixed wavelength detector (254-nm) based on a low-pressure mercury vapor lamp. The instrument did not fit into Picker Nuclear’s range of non-chromatographic products and, shortly after, it was acquired by Varian Associates (Walnut Creek, CA).
Almost simultaneously, Waters Associates – a leader in size-exclusion chromatography at the time – modified its integrated GPC-100 instrument to perform regular HPLC. Changes such as reduced dead-volume, a higher-pressure Milton Roy pump, as well as a flow-through UV detector and (optional) refractive index (RI) detector were incorporated into the existing instrument. The ALC-100 (see Figure 3) was introduced at the 1968 Pittsburgh Conference (ALC was an acronym for analytical liquid chromatograph). Waters immediately became “The Liquid Chromatography People” and remains a leader in HPLC today.
Dupont, the Delaware-based chemical giant, also joined the unfolding HPLC (now high performance LC) market. Introduced in 1969, the Model 820 integrated chromatograph (see ‘Honorable Mention’ 1b) had a constant pressure pump, a home-grown UV 254-nm detector (Model 410) and their own ZIPAX SPP. They introduced the first chemically-bonded phases that revolutionized the practice of gradient HPLC. Their ZORBAX column products remain and are now manufactured and sold by Agilent Technologies. (Dupont left the HPLC instrument market in 1986 followed by IBM Instruments, who briefly entered this market.)
History in the making
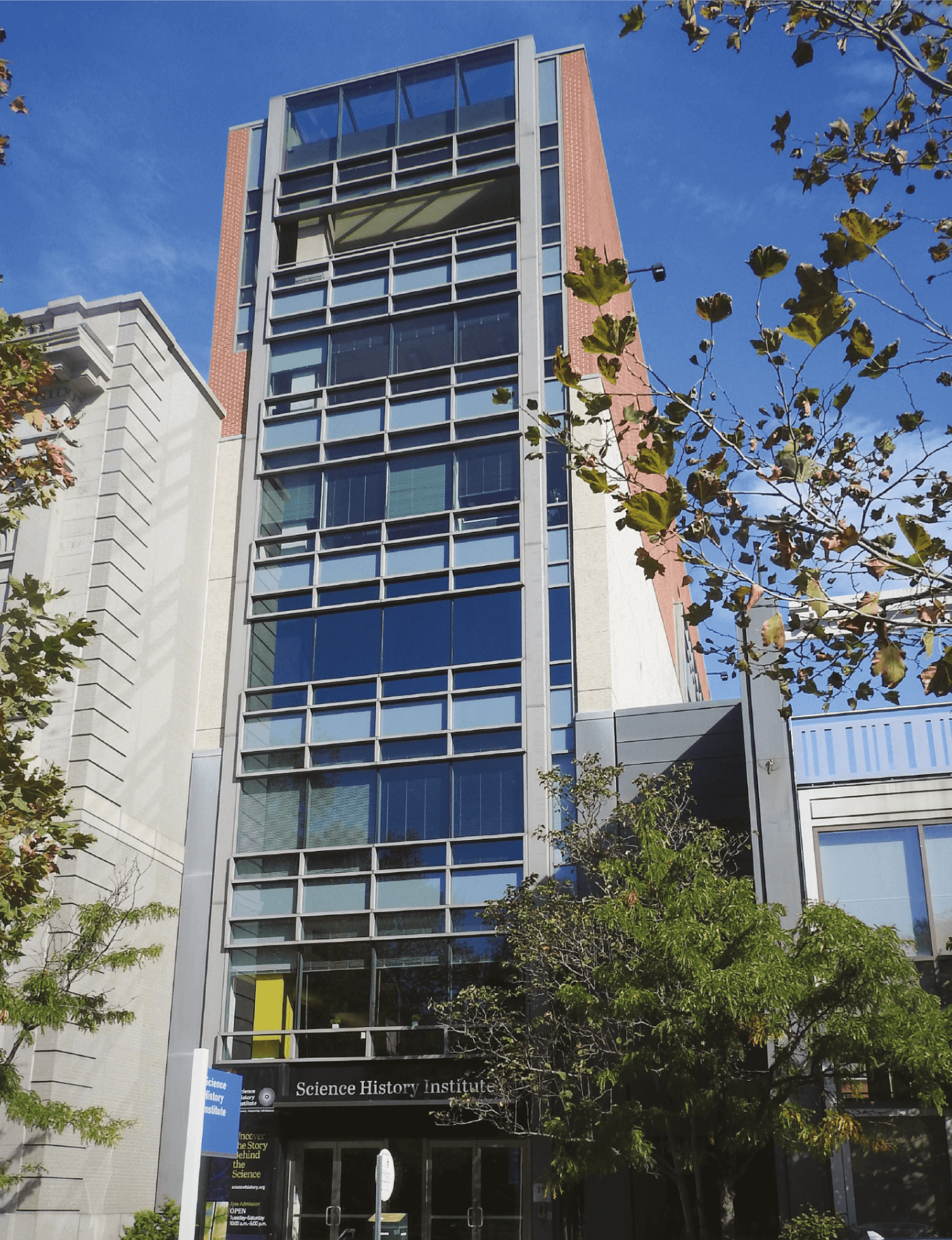
Within the Science History Institute, near Independence Hall in Philadelphia, you will find a permanent exhibit titled Making Modernity, which places historical analytical instrumentation in the context of the great human adventure of discovery in the chemical and molecular sciences. Other objects in the Institute’s collection are documented online at the Institute’s website (www.sciencehistory.org), while a large portion resides in storage, where it is available for use in rotating exhibits at the museum.
Much of the early instrumentation collected by the Institute originated from the Antiquities Museums of Pittcons 1994 and 1999. But the collection has grown over the years thanks to many donations, including a major one (133 instruments!) from Bodenseewerk Perkin-Elmer in Germany. To curate the collection, the Institute has relied on a volunteer group of active and retired chemists, who formed an Instrument and Artifacts Committee (IAC) in 2000 to provide guidance on what instruments to collect, what donations to accept, and to offer input towards the design of the permanent exhibit.
The IAC debated and then published a list of “50 Instruments That Changed the World” in 2004 and followed that with another “10 Most Wanted” list of instruments that were actively sought by the museum. Both lists were updated periodically as debate on the former continued and some, but not all, most-wanted instruments were acquired.
Today, the IAC has transitioned from creating lists to debating how best to identify and collect instrumentation that adequately documents advances across all fields of chemistry as the present becomes the past. Limited storage and display space is one challenge, but the Institute occasionally receives offers to acquire historically-significant instrumentation that is simply “too large to collect!”
In truth, the current collection is somewhat skewed towards spectroscopic instrumentation (the majority of IAC members were spectroscopy specialists in the early days, after all!). The chromatographic collection includes some early GC instruments, such as the F&M 700, P-E 154, and a Varian Aerograph 2100 among others, but we have only a limited selection of HPLC instrumentation. And that’s why we are keen to collect or document instruments that have “made a difference” in the 50 years of development of the liquid-phase separation technology.
You could consider the technology featured in the “Top Ten” and “Honorable Mentions” our wish list! If you can help contact: [email protected].
Injecting some sense
Fortunately, when packing the new SPP packing materials (37-50-μm particle diameter range) introduced by Dupont and Waters (3) into standard columns (2.1 mm ID, 50- or 100-cm length) the back pressure was modest, so the first commercial liquid chromatographs employed an on-line GC septum injector. As the packing material particles became smaller, the pressure capability of septum injectors was exceeded, spurring the use of stop-flow techniques. Such manual injection was cumbersome, limiting sample throughput, so Stan Stearns, founder of Valco (Houston, TX), adapted his GC valve to allow injection pressures up to 4500 psi. The six-port injection valve (see rank No.4) was a real breakthrough in productivity and reproducibility and allowed flexibility in sample volumes by changing the sample loop size. It also allowed later automation with autosamplers.
Waters introduced their U6K Injector (1973) after using a six-port injection valve in their integrated LC series (see ‘Honorable Mention’ 2a). It offered convenient, variable volume and reliable injection and could be automated. The injector also possessed an innovative bypass channel that reduced the pressure shock when the valve was cycled, which protected the column against collapse after repeated injections. Columns were less robust in the early days but as column packing methods improved it became less of a problem.
Later, Rheodyne’s (Berkeley, CA) Model 7125 Injection Valve (1976), modified the Valco approach such that the syringe loaded the sample into the center of the valve allowing variable injection volumes using a single loop (see ‘Honorable Mention’ 2b).
The rise of the module
In the early days, many chromatographers reasoned that each manufacturer had different strengths and therefore wanted to couple the best pump with the best injector, and the best detector, and so on. The aim was to build a superior system with quickly interchangeable (or upgradable) modules. To meet the demand, some manufacturers (especially those in the OEM business) decided to develop standalone modules that could be optimized for performance. The first of these companies was Laboratory Data Control (LDC, Riviera Beach, Florida) who also employed the services of Emmett Watson to build reasonably priced standalone modules, including a 254-nm UV detector (see rank No.3). This detector became the workhorse OEM component for several chromatography companies. The much larger Milton Roy Company acquired LDC and became a supplier of modules to many HPLC companies and individual chromatographers. Component companies like Valco and Rheodyne specialized in injection and switching valves. Detector companies like Schoeffel, Cecil Instruments (honorable mention) and Pye Unicam developed specialized detectors. Many smaller companies developed other peripherals that could be quickly exchanged as the need arose.
Pump it up
Waters, hopping on the module bandwagon, developed a standalone pump called the M-6000 – the first expressly developed for HPLC (see rank No.4). Early pumps based on a one piston design delivered pulsating flow, resulting in the need for pulse-dampening systems that could reduce noise in the flow-sensitive detectors. However, the large volume of pulse dampers delayed gradient mobile phases from reaching the column, increasing analysis times. The M-6000 used two reciprocating pistons for smoother flow to the column. And its pressure rating of 6000 psi was sufficient for the 10-μm microparticulate packings in 25 cm columns (4.6 mm ID) introduced in the early 1970s. Altex (Berkeley, CA) developed an entirely new concept using a variable piston speed with fast refill (see ‘Honorable Mention’ 4b); the flow from the resulting Model 110 pump was smoother than most low-cost reciprocating pumps of the day.
To provide higher pressures with non-pulsating flow, Varian Associates (Palo Alto, CA) (see ‘Honorable Mention’ 4a), Isco (Lincoln, Nebraska) and Nester-Faust (Norwalk, CT, later Perkin Elmer) chose to develop syringe pumps. Here, large volume (250 mL) pistons were driven by a precise stepping motor. These pumps were basically pulse-free and could reach high pressures, up to 6000 psi and later 8500 psi. For binary gradients, two syringe pumps were required. But chromatographers ran into problems during gradient elution because of solvent bulk compressibility; the actual flows of each solvent to the mixer were not as programmed on the controller, generating compositional errors – especially when each pump had a different starting volume. Syringe pumps, though unique and novel, eventually vanished. Nevertheless, syringe pumps deserve an honorable mention since this novel technology attempted to think outside of the box in providing pulseless, high pressure flow.
We Couldn’t Have Done It Without You
The authors sincerely thank experts in the field of HPLC, mass spectrometry, and data systems for lending their time to help identify the real breakthrough instruments, supplying dates of product introductions, and helping to run down information that has long been “lost,” especially in the commercial sector.
John Dolan (retired, LC Resources), Bob Stevenson (columnist emeritus, American Laboratory) and Dick Henry (founder, Keystone Scientific) spent countless hours digging through their files (and basements) to come up with some key contributions.
Tom Jupille (retired, LC Resources), Jane Gale (Historian at the American Society of Mass Spectrometry), Jack Henion (Emeritus Professor at Cornell University and Founder of Advion Biosciences), Dieter Hoehn (retired VP, Hewlett Packard), Geoff Cox (retired HPLC expert) and Glenn Ouchi (retired data handling expert) deserve thanks for helping to run down some critical information and provide sources of materials.
Hello, Industry 3.0
In the late 1960s and early 1970s, strip chart recorders were the main data output. For quantitation, manual methods, such as cutting and weighing the chart paper or using a mechanical planimeter, were the norm. But this all changed when Autolab, later a division of SpectraPhysics (Sunnyvale, CA), introduced their System IV computing integrator, which provided digital readout. Output could be presented as simple area percent or based on calibration factors stored in the method. Because of its large dynamic range, chromatographers no longer had to make multiple injections while adjusting the signal attenuation, which increased laboratory productivity. The microprocessor-controlled Hewlett Packard HP 3380A integrator (1974) (see ‘Honorable Mention’ 5a) went further and served both as a recorder and alpha-numeric printer-plotter so all information was on a single piece of chart paper. After that, many manufacturers introduced their own data systems to supplement the HPLC hardware. Finally, Nelson Analytical (1979) developed data analysis software based on personal computers. They took advantage of new-large scale integrated (LSI) circuitry to construct analog-to-digital converters and provided instrument control and data acquisition with powerful calculation ability. The Nelson products became the standards in systems of many manufacturers. When the IBM PC was introduced, Nelson adapted their software to allow both instrument control and data handling – a concept that quickly replaced the standalone integrator in future instruments.
About the same time, in the mid-1970s, HPLC was increasingly being used in pharmaceutical and other industries, where users needed to analyze many samples per day. The first HPLC autosampler to reach the market was from Georgia-based Micromeritics, a company specialized in particle size measurement. The Model 708A LC Autosampler (1974, see rank No.5) used tubular vials in a rotating tray. A needle was lowered into the vial, puncturing its cap, while a collar simultaneously pushed the cap down into the vial displacing the sample into the sample loop. The system allowed 1-3 injections from the vial. By the end of the 1970s, most of the major suppliers had introduced their own autosampler.
Revenge of the integrated system
As HPLC was experiencing widespread acceptance, central control of instrument peripherals made better sense; the component LC concept was losing favor as modules couldn’t all talk to each other. Users also discovered that gradient elution was a must for analyzing samples with a wide variety of components, which acted as another driving factor towards central control. Two-pump gradient systems for binary gradient production were the first type designed (the two pumps were plumbed together on the high-pressure side and a mixer was required).
Hewlett-Packard’s Analytical Division (now part of Agilent Technologies) improved on the Hupe-Busch system that they acquired two years earlier. The HP 1084 (see rank No.6) was the first microprocessor-controlled LC with unique flow control plus an autosampler, UV detector, and an external fluorescence detector. The precise flow control of the 1084 was a great selling feature and gave highly reproducible retention times.
Since two-pump gradient systems were expensive to build (and buy), developers such as Varian and Spectra-Physics worked on the early products that used a single pump with solvent proportioning valves on the low-pressure side. Thus, with two- or three- proportioning valves, users could generate binary or ternary gradients, offering more flexibility during method development. Low pressure gradient formation eventually became the standard for many companies. Later, even more powerful quaternary pumps were developed. The Varian LC-5000 (1978, see ‘Honorable Mention’ 6a) was the first integrated HPLC system that incorporated low-pressure single pump gradient capability, microprocessor control of key features, a keyboard for setting up methods, and a CRT display all in a single unit. An active inlet check valve solved the problem of sticky check valves and loss of prime. The Spectra-Physics Model 8000 launched in 1979 employed a design that is almost identical to the solvent blending designs used in today’s instruments.
In 1975, Dionex was carved out as a division of Durrum Instruments to address an emerging chromatography market: ion chromatography (IC). Dionex quickly became the leader in separation of inorganic and organic ionic compounds (see ‘Honorable Mention’ 6b). Their patents, licensed from Dow Chemical, covered the use of an ion suppressor to remove salts from the mobile phase, which allowed conductivity detection of the separated ions. They also developed specialized IC columns that were devoted to tough separations, such as trace bromates and oxyhalides in drinking water, carbohydrate separations using pulsed amperometric detection, and ion exclusion separations. Now owned by Thermo Scientific, Dionex still controls the IC market.
Sophistication in detection
In the first 10 years of HPLC, spectroscopic detectors dominated. Stop-flow scanning spectrophotometric detectors met with little acceptance in the marketplace. With the adoption of optical diode arrays, real-time spectroscopic measurements could be performed and complete UV-VIS spectra could be obtained on-the-fly, which became the industry standard. HP’s 8450 Diode Array Detector was the first product to market (1977) using this technology, and it set the gold standard for diode array detectors of the future.
Following the remarkable success of GC-MS, various interface types were developed to combine two apparently incompatible techniques – one in a liquid environment and the other in a vacuum. Interfacing technologies included direct liquid interfacing, transport devices, particle beam, continuous flow FAB, ion spray, and thermospray.
The first in the marketplace was the moving belt interface (a transport interface). The technology was borrowed from early work by R.P.W. Scott (4) and Victor Pretorius (5), who used a moving wire to transport part of the LC column effluent to a flame ionization detector (marketed by Pye Unicam in the UK). Rather than a moving wire, the first successful LC-MS interface used a moving belt which allowed more sample to be transferred for better sensitivity. Solvent was evaporated by heat prior to entering the vacuum zone of the MS. Finnigan MAT introduced this interface in 1976 (6). On the plus side, the interface offered good EI spectra and good sensitivity. But disadvantages included difficulties with non-volatile labile analytes and LC buffers, and unreliable performance.
Enter Thermospray, a more reliable soft ionization interface that passes the column effluent through a very thin heated column to produce a spray of fine liquid droplets. The droplets are ionized at atmospheric pressure (API) via a low-current discharge electrode to create a solvent ion plasma. EI-type fragmentation is sometimes observed but there is significant fragmentation of protonated or deprotonated molecules. Sensitivity is low for non-volatile analytes. Developed by Marvin Vestel (7), who formed Vestec to commercialize the interface (1987), Thermospray was the most used interface until the 1990s and certainly deserves a special mention.
The electrospray interface (ESI), developed by Nobel laureate John Fenn while at Yale University (8), produces intact, high-molecular weight, multiply protonated or deprotonated ions. Jack Henion and colleagues (9) developed an ion spray interface that used nebulized nitrogen to assist electrospray operation; Sciex, using the Henion patent (10), was first to market with an ESI-type interface (1989, see ‘Honorable Mention’ 7b). Along with various other API techniques, ESI is now the standard LC-MS interface.
Other universal detectors were developed. The evaporative light scattering detector (ELSD) and the charged aerosol detector (CAD) both nebulize the LC mobile phase effluent into droplets, which are evaporated leaving behind small particles of non-volatile analytes. In general, the CAD, first introduced by ESA Biosciences in 2005 (see rank No.9) is more sensitive than the ELSD and can be more gradient friendly. The CAD can also detect all non-volatile and many semi-volatile analytes with a uniform response.
Age of ultra
Through most of the 1980s and 1990s, liquid chromatographers were happy to use 3–3.5 μm or 5 μm particles, 6000 psi pumps, and 4.6 mm ID columns. However, to meet the need for faster analyses, packing materials with sub-two micrometer dimensions were developed. Once again, systems were pushed to higher operating pressures. And, because the resulting viscous heating effects required smaller diameter columns, a significant reduction in extra-column volume was also necessary. The Waters Acquity UPLC system (see rank No.10) was designed to meet the needs of the new small-particle shorter columns (5 to 15 cm) with narrower bore (2.0–2.1 mm ID) in the early 2000s. The timing was right in that Acquity was a thoughtful combination of existing products/technologies resulting in a practical system with reasonably low extra-column volume. The innovation inspired other companies to make similar improvements to benefit from the increased efficiency of newer columns. Thus, a new (non-trademarked) nomenclature arose for these ultra-high-performance instruments: UHPLC. Today, over a dozen companies are in the UHPLC business.
What about the next 50 years?
We asked our expert panel to gaze into their crystal balls and predict at least one game changer they’d expect to see in the next 50 years of HPLC
Majors: In the short-term? I’d expect to see miniaturized easy-to-change monolithic or pillar columns fully integrated into the handheld – or pocket sized – battery powered LC system without end fittings or added dead volume. In the long-term, I think we’ll see miniaturized, personal separation systems with integrated components and sensitive, selective universal detection capable of performing real-time analysis of a person’s health status.
Jupille: I think we’ll see a change to “instrument as an appliance,” with a one-button “Analyze This!” interface. Longer term, I hope powerful AI-based systems integrated with LC hardware will make “walk-up analysis” a reality: The analytical chemistry analog of a self-driving car.
Dolan: A dedicated analyzer with integrated sample preparation. The sample could be deposited on card or finger-prick, inserted into instrument and produce complete analysis (for example, drug screening) in less than a minute. It would also be great to see inexpensive continuous/periodic monitoring LC devices that predict breakdowns before they happen!
Stevenson: LC coupled to an ion mobility spectrometer in a wrist-watch configuration, where the watch circumference provides the dimensions of the drift tube. But I also predict some application drivers in the next 50 years: wellness checking – “match the drug to the bug” – or point-of-sale freshness indicators and confirmation of identity.
Hoehn: I reckon we’ll see a hand-held product for general use in life sciences.
Ouchi: In the next 50 years? I think the user will be able to submit a sample to an instrument, select the analyte(s) to find in the sample and a smart instrument will automatically analyze from an inboard list.
Henry: In the short term, I believe we could see a practical version of a multidimensional LC system where current problems in quantitation are solved. In the long term, smart, automatic instruments where an unskilled user does not have to change columns, develop methods or perform quantitative analysis.
And a few more innovations that helped the practitioner...
Dissolved air in solvents was a pain for early HPLC. Gas in the mobile phase caused pump cavitation and loss of prime. Gas in the mobile phase at the exit of the column caused bubbles in the detector flow cell. Degassing solvent was required, first attempted by boiling the solvents and trying to keep the gas out by cleverly designed reservoirs. Then along came SpectraPhysics, who showed that helium sparging of the reservoirs kept air from dissolving in the solvents and allowed low-pressure mixing to be used effectively for HPLC pumps. Later on, membrane degassers replaced helium sparging and they became a part of every HPLC/UHPLC still used today.
Fittings also proved to be a headache. More often than not, chromatographers would over-tighten fittings giving rise to potential leaks and poor performance in trying various reconnections. Fittings from different companies were incompatible and dead volumes were created. Upchurch Scientific’s Fingertight fittings changed all that and made easily reused connections that, even today, can handle very high pressures.
The year 2020
(U)HPLC is now the most widely used separation technique. The early milestone developments in the initial phase of its 50 plus years of existence paved the way for the sophisticated instrumentation and columns that we have today. The technique continues to evolve with refinements that include miniaturization, LC-MS/MS, and two-dimensional separations (and beyond), leading us toward the extremely high peak capacity required for the complex samples encountered today. Separations that early workers could only dream about have come to pass and, in many cases, are routinely used in laboratories throughout the world. The sensitivity and selectivity of today’s detectors blow the minds of those of us who grew up with the 254nm UV detector of the 1960s. And separation times of less than a few seconds have been achieved by researchers who used to struggle to get their separations times under an hour… What a ride!
Does your Top 10 match ours? We’d love to hear your thoughts (or even grievances) and urge you to share them in the comments section below!
References
- CG Horvath, BA Preiss and SR Lipsky, “Fast liquid chromatography: an investigation of operating parameters and the separation of nucleotides on pellicular ion exchangers”, Anal Chem 39, 1422 (1967).
- CH Arnaud, “50 years of HPLC”, Chem Eng News 94, 29 (2016).
- JJ Kirkland, ed., “Modern Practice of Liquid Chromatography”, John Wiley and Sons (New York, 1971)
- RPW Scott and JG Lawrence, “An improved moving wire liquid chromatography detector”, J Chromatog Sci 8, 65 (1970).
- V Pretorius and JFJ van Rensburg, “Improvements to the wire solute transport detector”, J Chromatog Sci, 11, 355 (1973).
- WH McFadden, HL Schwartz and S Evans, “Microbore high-performance liquid chromatography mass spectrometry”, J Chromatogr 122, 389 (1976).
- CR Blakely and ML Vestel, “Thermospray interface for liquid chromatography/mass spectrometry”, Anal Chem 55, 750 (1983).
- JB Fenn et al, “Electrospray Ionization for Mass Spectrometry of Large Biomolecules”, Science, 246, 64 (1989).
- AP Bruins, TR Covery and JD Henion, “Ion spray interface for combined liquid chromatography/atmospheric pressure ionization mass spectrometry”, Anal Chem 59, 2642 (1987).
- JD Henion, TR Covey and AP Bruins, Ion Spray Apparatus and Method, United States Patent No. 4861998 (1989).