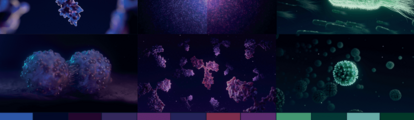
Talkin’ ‘Bout a (Protein) Revolution
How native MS may help rewrite the textbooks on immunology – and life itself
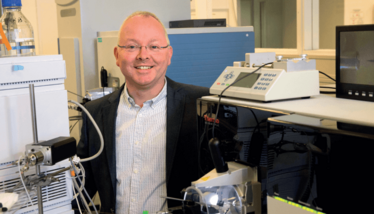
For researchers in the life sciences, the “protein revolution” happened quite some time ago. But for the general public, proteins are still somewhat of an alien concept – unless you’re talking about counting your “macros” as part of the latest dieting trend. Though most people are well aware that DNA carries genetic information and that this has an effect on our lives – from the color of our hair to our predisposition to certain diseases – most do not fully understand the vital role proteins play in every living process.
One of the few positive things to come out of the COVID-19 pandemic is that people have (in general) gained extra understanding around concepts like viruses, antibodies, and vaccines – and, in turn, the action and function of proteins. As scientists, we should welcome the public’s newfound (non-literal) appetite for proteins with open arms – not only is it a sign of how far we’ve come, but of how much there is still left to explore and understand.
As we learn more and more about our proteins, scientists around the world are making fascinating discoveries. My own team recently uncovered evidence that we are even more unique at the proteome level than was ever previously thought – and that this can explain a lot about how and why we all react so differently to diseases, drugs and vaccines. But more on that later…
First, I hope to bestow upon you all the same passion I have for native mass spectrometry – an exciting technique that could help us write the next chapter in the protein revolution.
Going native
So what is native mass spectrometry? Native MS is a term that we initially coined here at Utrecht University. (There’s a similar well-known technique in biochemistry called native gel electrophoresis, where non-denaturing gels are used to analyze proteins and protein complexes in their folded state – and that’s partly where the name came from…)
In contrast to traditional MS, with native MS we try to keep the structures of the proteins and protein complexes that we analyze as close to what they were in their native environment of the cell. To do this, we use special solvents that not only maintain the integrity of the protein interactions, but are also compatible with the electrospray ionization process that transfers molecules from the liquid to gas phase. By using this unique set-up, it means even non-covalent complexes can remain intact for analysis. In the words of the Nobel Laureate John Fenn, through electrospray under these pseudo-physiological conditions, we can make elephants fly (1).
By keeping proteins and protein complexes intact in the mass spectrometer, we’re able to accurately measure the masses of interesting biomolecular machineries, such as intact antibodies, viruses or ribosomes. It also allows us to confirm the composition of these systems; for instance, do we have a monomer or tetramer (the tetramer will have a mass that is four times as great as the monomer). Even when a protein complex consists of about 20 different proteins and RNA; as for instance in an intact ribosome, with a mass close to two million Daltons, we are able to accurately measure its mass in a native state and observe changes in its composition.
Accuracy is the real key to native MS. After all, we are only measuring the masses of the complexes, but we do it so accurately that we are able to learn about their composition and whether this changes over time – like when they exchange or add new subunits. And that means we’re also able to learn a lot more about the function of these macromolecular complexes. For example, a change like a phosphorylation or glycosylation, which also induces changes in mass, may either activate a protein or inhibit a protein’s function. By discovering whether a protein is modified or not, you can learn how to activate or deactivate a protein. In turn, this means you could aim to regulate entire biological processes. And that’s just one reason why measuring these masses so accurately is important – there are also many other incredible applications (see for instance our work on a bacterial biological clock).
Gene therapy
When my team and I first started using native MS, we looked at virus-like particles (VLPs). VLPs resemble viruses; they form beautiful, very rigid spheres made up of self-assembling proteins – this is known as the capsid. However, these VLPs lack the genetic information needed to infect a host cell, making them safer to analyze. On the other hand, intact or “native” viruses contain not only the capsid, but also all the genetic information that enables the virus to reproduce within the host. One well known example of a native virus – and one that is not harmful to humans – is the adeno-associated virus (AAV). In recent years, biopharmaceutical companies have used AAV as a vector for delivering certain genes into human cells by replacing part of the viral genome – the basis for gene therapy. A notable example of this is Zolgensma; a prescription approved AAV vector-based gene therapy for the treatment of children less than 2 years old with spinal muscular atrophy introduced by Novartis.
Even more recently, adeno-virus (ADV) vectors have garnered attention because of their use in the AstraZeneca, Janssen and Sputnik vaccines for COVID-19. Without a doubt, these gene-loaded viral particles are an exciting class of emerging biopharmaceuticals. However, they are also extremely complicated to produce and structurally very heterogeneous and therefore raise huge and specific novel analytical challenges.
We work with several pharmaceutical companies to help them overcome some of these challenges by analyzing virus-based gene delivery vectors using native MS. Why do they need our help? First of all, it’s about sensitivity. It’s very hard and laborious to produce these particles, so you never have many of them available to analyze – unlike recombinant antibodies where you can produce grams. There are also further complications because these particles are huge – we are talking 4–5 million Dalton. And they are extremely heterogeneous, containing several different proteins and gene products, which makes them difficult to analyze with a single technique.
We pioneered the use of a particular type of native MS – charge detection MS – for this exact purpose (2). This technique’s big advantage is its ability to count, while accurately measuring the mass of, single viral particles. And it’s really opened up a whole new avenue of analysis, allowing us to look closely at gene vectors and discern whether or not the genome of choice has been successfully loaded. Understanding whether the gene has been incorporated is vitally important for the biopharmaceutical companies producing these particles. As such, I foresee that native MS will play a crucial role in the quality control of gene delivery factors for this exciting new field of medicine.
Rewriting the textbook on immunology
More recently we also started to focus on antibodies, as they are naturally present in huge quantities in our blood. According to the textbooks, antibodies are made by certain cells in our body called B cells. The number of different B cells in our body is enormous – we’re talking 1015 , which to put it in perspective is several times more than the number of people on the planet. I had been wondering myself already for some time: how many (and which) antibodies does the human body really produce that end up actually circulating in our blood to counteract invading pathogens? The commonly accepted answer within immunology was that this could be an almost infinite (or at least, immeasurable) number. When we suggested measuring them all, many within the field thought it would be impossible. We’ve now published research that proves the task was not as insurmountable as people thought (3). To our surprise, we found that just a couple 100 different antibodies dominate the repertoire in each person’s blood – a number so low in comparison to what was previously believed, that we knew these findings were already very exciting. But then we also discovered that each person had their own personal repertoire of antibodies – equally exciting.
You might be thinking that such a finding is somewhat obvious; “Of course we would make different antibodies against every virus or pathogen we encounter,” you say. “I’d expect there to be a unique signature to align with this fact.” However, we observed that, even if people are exposed to exactly the same pathogen – or vaccine in the case of COVID-19 – they still make their own personal repertoire of antibodies against it. Fascinating. This clearly implies that every person reacts to incoming pathogens differently. We can see this working on a grand scale when we look at how everyone has reacted to the COVID-19 vaccines; some people get ill, others don’t, and the vaccine works better at protecting some people from the virus than others. We now know that these differences are at least partially due to the specific antibodies each person makes. (For more information, see the sidebar: What’s the deal with antibodies?)
In the long run, I hope that we will be able to measure and identify the antibodies that each person makes against a certain pathogen using MS. This would open up the possibility of taking antibodies from someone who does mount a good response, and using them to help those who don’t. In this case, we’d be using recombinant molecular biology to produce the best antibodies against the disease and treat someone who has a sub-par response. Such treatment could be used against all COVID-19 variants, but I’m equally eager to see how this could be applied to any other viral or bacterial infection – or even other diseases, such as rheumatoid arthritis and cancer. Basically, new understanding in this arena could be applied to any disease to which our bodies respond with antibodies – and that’s quite a few! Ultimately, it could open a new way of producing personalized biotherapeutics.
Our finding was extremely exciting and totally unexpected – and one that is bound to find its way into the textbooks in the coming years. It’s not often you get to make such discoveries, so I’m extremely proud that our team of about ten researchers, who worked on this for a couple of years, was able to achieve this.
The horizons of MS
MS has come a long way since its inception more than 100 years ago, but some challenges seem to always remain the same. Can we get faster? Can we get more sensitive? Can we get higher mass resolution? Can we get better mass discrimination? Though many great advances have been made, the answer to all these questions is yes, we could and should. It’s incredible to think that we now only need tiny amounts of material to run our analyses, which means we can uncover the entire proteome of a single cell. This is an enormous sensitivity jump from where we were. However, the cell is still made up of billions of proteins. The area I’m most excited about for the future – and an area we’ve also contributed to ourselves – is in increasing the sensitivity of MS to measure single molecules.
My ultimate dream is to take a single cell, take out every molecule one by one and measure its mass. And though it might sound like a pipe dream, it’s already theoretically feasible. Practically, I’m not sure when we’ll manage it – but, because I can imagine how it might happen, I’m sure someone will figure it out soon enough, and of course we hope to contribute to that.
For me, progress is all about understanding, fundamentally, how life works. In turn, we increase our knowledge about our wellness, health, and the planet. The horizons of MS are already beautiful. But beyond that horizon, there are undoubtedly new and wonderful stories – applications that many of us haven’t even dreamed of yet.
Albert Heck will be presenting his talk ‘New Horizons in Mass Spectrometry: Sizing and Counting Particles by Native Mass Spectrometry’ at the 2022 Wallace H. Coulter Lecture at Pittcon. Learn more here
What’s the deal with antibodies?
Our data shows that, when you receive a COVID-19 vaccine, your body doesn’t just make one antibody against the spike protein – it makes 10–20 different antibodies in what’s called a polyclonal response. Now, most of these antibodies are probably pretty good at their job – blocking the spike protein and stopping the virus from entering host cells. However, if a new variant pops up, it’s a different story; for example, out of the 12 antibodies that were initially effective, six may not be effective against the new variant. By producing a range of antibodies, your body is better prepared for any changes (mutations) the virus might undergo – and this is partly why we think our bodies don’t just produce a single antibody.
In other words, a polyclonal response helps prepare our bodies for the unexpected. I sometimes like to think about a theoretical question: What would happen if a pathogen landed on Earth from somewhere else in the universe? Would it wipe out the human species? Or would we be able to harness the power and flexibility of our immune system to create antibodies against something so very foreign? Clearly, I have no concrete proof, but I think the answer lies closer to the latter. I think at least some of the population would be able to mount a good response against such a pathogen.
We’ve managed to survive a long time on planet Earth thanks to the millions of years that have been spent designing a complex and beautiful immune system. And modern research is still only scratching the surface.
Image credit: SENSU
- JB Fenn, Angewandte Chemie (2003). DOI: 10.1002/anie.200300605
- TP Wörner et al., Nat Methods, 17, 395 (2020). DOI: 10.1038/s41592-020-0770-7
- A Bondt et al., Cell Systems, 12, 1 (2021). DOI: 10.1016/j.cels.2021.08.008
Albert J. R. Heck is a professor at the Science Faculty of Utrecht University in The Netherlands. Heck is also scientific director of the Netherlands Proteomics Centre and coordinator of the European proteomics infrastructure PRIME-XS and the NWO roadmap funded Proteins At Work. “I’m interested in implementing innovative mass spectrometric methods, with an emphasis on protein interactions and post-translational modifications,” he says.