Analytical techniques were crucial to the genesis of chemistry as a field, and today they continue to be essential in driving advances not only in the understanding of chemistry, but throughout all scientific disciplines. Despite this continued importance, analytical chemistry is suffering from an identity crisis. Though still the most common field of employment for chemistry graduates in industry (1), analytical chemistry in academia is losing impact and funding, and the field is poorly represented amongst top research institutions. One of the reasons for this is that modern analytical chemists take many guises. Analytical chemistry departments are well-stocked with those engaged in forensic analysis, or electrochemical studies, but there are many others who would not label themselves as analytical chemists, yet engage in the development and application of analytical chemistry. Though analytical chemistry itself remains best placed to address many contemporary challenges – from environmental changes to the study of disease – it is important that all researchers involved in the field work see that powerful analytical tools are developed and used to greatest effect.
In the biosciences, those who develop and use analytical tools might now brand themselves ‘chemical biologists’ or ‘biochemists’, but they are engaged in the age-old analytical problem of identifying and quantifying chemical species. In this field, as in other areas of science, it is clear that analytical chemistry has much to contribute, but how can we ensure that bioanalytical research can achieve its highest impact? Here, we consider how analytical chemistry can be best harnessed for study of the biosciences, but our arguments could be applied to any scientific discipline.
The impact of analytical tools for the biosciences
We can think of the human body as a concentrated (and extremely complex) mixture of chemicals – protein, DNA, lipids, metal ions, and so on. To understand this system, we need analytical chemistry. Many of the key techniques that have driven biological advances have involved analysis, and this continues to be the case. The ‘omics’ revolution, which has given us genomics (analysis of the structure and function of an organism’s DNA), proteomics (the study of proteins, and their structure and function), and metabolomics (investigation of the breakdown products of cellular activity), amongst others, relies heavily on analytical chemistry techniques. For example, genomics relies heavily on DNA microarray technology; proteomics on mass spectrometry; and metabolomics on gas and liquid chromatography techniques. In general, the contributions of analytical chemistry to biosciences fall into three categories:- Technology – instruments that enable the identification and measurement of chemical and biological species. Within mass spectrometry alone, instruments, such as laser ablation-inductively coupled plasma-mass spectrometry (LA-ICP-MS), can give spatial resolution with sensitivities down to low parts-per-trillion, and high-resolution mass spectrometers can characterize the precise chemical makeup of large biomolecules. High-throughput DNA screening is a laboratory standard, and gas chromatography continues to thrive, even though its roots are firmly planted in the mid-1900s.
- Reagents – chemicals that can be used alongside technologies to enhance the information that can be gained from analytical technologies. By way of example, the enzyme-linked immunosorbent assay (ELISA) is a powerful technology for quantifying specific proteins in cell lysates, but its utility relies on the availability of a number of key reagents – the specific antigen or antibody, the secondary antibody linked to an enzyme, and the colorimetric or florogenic enzyme substrate.
- Protocols – where existing technologies and reagents are being repurposed in new and exciting ways. A prime example is the continued use of gas chromatography, which is being constantly redesigned, improved and modified to suit the emerging needs of multiple disciplines, from the environmental sciences to medicinal chemistry.
Perhaps because it has become invisible in its ubiquity, analytical chemistry is not at the forefront of the conversation regarding the future of the biosciences. Though there is no doubt that without key analytical techniques, far less would be understood about the omics, the challenge for the new generation of analytical chemists working at this interface is to not only re-enter this discussion, but to lead it.
It is important, at this point, to define the impact for which we should strive in this area. In academia, the emphasis on metrics regarding impact can cloud the matter (see below). Rather than seeking high impact factors, for example, analytical chemistry should instead strive for real-life impact. Since the greatest contributions of analytical chemistry to the biosciences lie in the development of technologies, reagents and protocols, enduring impact can be measured by the uptake of these developments by other researchers.
Achieving impact
There are a number of simple ways in which analytical chemists working in the biosciences can ensure greater impact.Asking the right questions
The questions of greatest biological significance are not always the easiest to answer, but they should direct future analytical efforts. Researchers involved in the development of analytical tools must not work in isolation, but must be constantly guided by those working at the forefront of medical research.Developing accessible tools
Though many biological questions can only be studied by the most sophisticated of technologies, a breadth of impact requires the development of tools that are accessible to a wide range of biological researchers. In other words, technologies that are not prohibitively expensive, reagents that can be used with routine technologies, and protocols that do not require highly specialized personnel for operation. The most impactful medical research requires simultaneous use of a broad range of analytical techniques, so highly specialized and expensive equipment for a single measurement will not be beneficial in advancing individual, or even consortia of research programs.Seeking valuable improvements and use of existing tools
There is a plethora of tools reported in the analytical chemistry literature that have never been used beyond their first report. Why? The lack of uptake (and impact) can largely be attributed to a failure to ask the right questions, but in some cases, results from the developer not knowing an appropriate biological question to probe. In this case, it is important not to re-invent the wheel – it could be that techniques developed for other fields, such as environmental sensing or even archaeology, could be readily applied to biological studies. Furthermore, the demands for ever-increasing sensitivity, specificity and resolution of research tools should guide efforts to improve existing tools.Demonstrating the potential impact of tools
There is a considerable inertia amongst the biological research community in adopting many new analytical tools. In general, it is preferable to adhere to tried and true methods, even if they are inferior, because biological research groups often lack the expertise to validate and troubleshoot new tools. To that end, the analytical chemists who developed the tools should take charge of such work. There will only be widespread uptake when tools are accompanied by rigorous data that demonstrate the reproducibility of results, identify any possible interferents, and show the breadth of systems to which the tool can be applied.Pursuing collaborations
All of the above points require that there is active cooperation between analytical chemists and biological researchers – at every step of the process. And in fact, seeking out new collaboration leads to more ideas for research questions to probe, and more biological systems in which to test new tools.Defining impact in academia
The term ‘impact’ has become somewhat corrupted in an academic sense – to the extent that it has lost meaning as a breadth or depth of effect, and is instead synonymous with ‘impact factors’ (a concept not understood or appreciated beyond academia). Impact in scientific literature is typically measured by the prestige of the journal in which a technique has been published, with journal impact factors being the main measure. Impact factors, released annually by Thomson Reuters’ Journal Citation Reports, are calculated by a simple formula that divides the number of citations a journal has received for articles published in the previous two years by the total number of articles published in that time period. Thereby, the higher the impact factor, the more perceived strength a journal carries. Impact factors are a controversial subject, with scientists working in niche disciplines often hamstrung by journals in their fields having low impact factors, which do not necessarily reflect the quality of the work or potential scientific impact it may have in years to come. Some government funding bodies now instruct reviewers not to consider impact factors of journals when assessing funding applications, though for many scientists this can be a case of telling them to ‘sit in the corner and not think about penguins’. Whether academic impact is measured solely on the basis of impact factors, or rather on a cruder measure of number of outputs, it is clear that these metrics play too large a role in driving research. In the contemporary academic world, the ‘publish or perish’ model remains alive and well, and it is therefore not unusual for analytical scientists to pursue impact within the scientific literature over real-world applications. It can easily be viewed as a flaw in the motivations of analytical scientists, though it is often the harsh reality in which analytical chemists live. New advances need dollars and, according to a 2013 report by Lab Manager Magazine and Frost & Sullivan, academic laboratories in the United States rely on public grants for nearly 50 percent of their funding. In the highly competitive grant system, the awarding of funding is often judged not only on the science, but also the previous impact of the researchers applying. Thus, demonstrating impact in the scientific literature is the most obvious route to securing funding for future projects.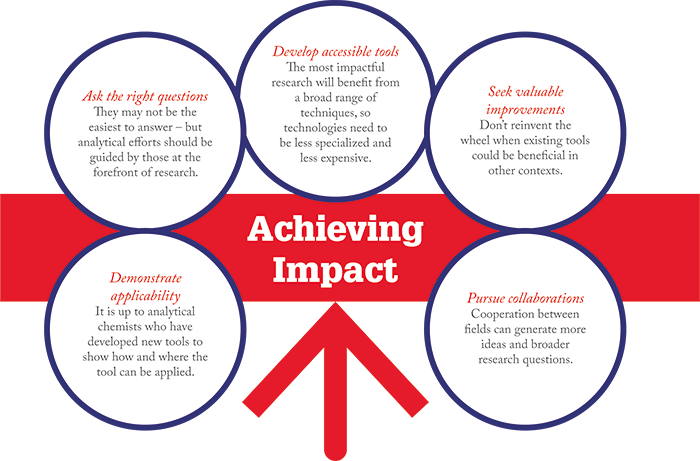
From a practical point of view, then, it is often easier to publish a minor modification to an analytical tool rather than to seek collaborations that demonstrate the breadth of applications of a tool that has already been prepared. Perversely, such an approach is at odds with the true impact of an analytical technique, which is defined by its uptake by a specific discipline. Although the old adage that ‘a craftsman is only as good as his tools’ is just as relevant to analytical chemistry, the finished product – or in this case the application – is the yardstick by which impact is typically measured. The disconnect between academic impact and practical impact arise from the typically long lag time between development of a new analytical method and its uptake within the targeted discipline. For the pure analytical chemist working in method development, it may be necessary to prioritize immediate academic impact over practical impact to secure funding in an environment where they are competing against scientists and researchers from multiple disciplines. Rather than demonstrating the real impact of a newly-developed technique, analytical chemists must rely instead on demonstrating the potential impact to funding bodies. It is noteworthy that two of only three papers to have been cited over 100,000 times were in the analytical biosciences field (2), clearly showing that impact can be a slow, yet highly significant process.
Defining practical impact
None of this should be considered a condemnation of the current state-of-play in academic research; rather, it should spur researchers to consider the five points listed above to actively pursue the best means for achieving impact by asking the right questions, developing meaningful collaborations, and designing accessible tools. On one end of the spectrum is the development of high-end and technologically complex systems, such as next generation mass spectrometers and super-resolution microscopes, which require significant capital investment that is usually provided by the private sector. However, the application of these systems is at the behest of the end user: analytical manufacturers may have application support personnel and active research and development divisions, but the work at the coalface is dictated by the ideas and needs of a particular discipline. The practical impact of these systems, which usually require significant capital investment, are often as significant as the development of the technology itself. One of the best strategies for achieving practical impact with immediacy is the integration of analytical sciences within the laboratories that seek to benefit from new developments. In doing so, not only does a method development chemist have direct access to the stakeholders they intend to engage with, these stakeholders can also convey the needs and importance that may not be immediately apparent to the analytical scientist.Modern bioscience is now focused on the molecular and chemical mechanisms that govern how a cell or organism develops, lives and dies. The shift of focus from the physical structure of a biological specimen that was made possible by the invention of the light microscope in the 1600s to the chemical makeup of a sample is inexorably tied to analytical methods. Some of the most significant advances in biology in the past few years are rooted in our ability to chemically observe molecular changes. For example, the CRISPR gene editing technique has huge potential in biology – everything from addressing antibiotic resistance to directing new gene therapies for cancer treatment – yet nothing would be possible without the ability to measure, monitor and detect outcomes of what is essentially chemical reprogramming of a gene. However, CRISPR was not made possible through a single analytical advance; decades of development and refinement of the fundamental methods for studying the chemical makeup of genes produced techniques that were essential for this revolutionary discovery.
A future role for analytical chemistry
So, does the analytical chemist have an identity crisis? In short, the answer is no. Analytical chemistry, like all scientific disciplines, is constantly evolving, and it is up to the chemists themselves to keep pace with the fast moving and dynamic needs of biologists. Perhaps the pure analytical chemist is a dying breed of scientist, but that should not deter those who work in such a space from embracing their redefined roles. Those who once made the tools are now also becoming the architects who help shape the new discoveries that are advancing the biological sciences – and researchers from multiple disciplines are becoming more reliant on the skills and knowledge possessed by the analyst. Those who can close the gap between analytical development and practical impact will shape the future of humanity. So, who are you – and how are your bridge-building skills? Elizabeth New is Senior Lecturer and Westpac Research Fellow at the School of Chemistry, University of Sydney, and Dominic J. Hare is Senior Lecturer at the University of Technology Sydney and Head of the Analytical Neurochemistry Development Group at the Florey Institute of Neuroscience and Mental Health at the University of Melbourne, Australia.References
- SL Rovner, “Salaries & Employment”, Chemical & Engineering News, 92, 68–71 (2014). R Van Noorden et al, “The top 100 papers”, Nature 514, 550–553 (2014).