Arrested Development
Given ongoing challenges in sample preparation and a lack of novel alternatives, is it time to give liquid-phase microextraction (LPME) a second chance?
Stig Pedersen-Bjergaard, Astrid Gjelstad, Knut Einar Rasmussen |
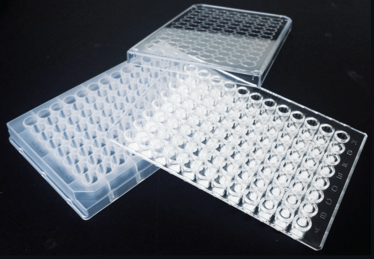
The Problem
Complex aqueous samples, such as biological fluids and environmental water samples, are a real challenge for sample preparation. Current methods are marred by poor sample clean-up, dilution of the sample, and use of solvents. The discovery of liquid-phase microextraction (LPME) in the late 1990s offered a welcome alternative – so why hasn’t the technology been commercialized?
Background
Liquid-phase microextraction (LPME) is a microextraction technique for sample preparation prior to chromatography, mass spectrometry, and electrophoresis – and it was first presented by our group in 1999 (1). The target analytes are extracted from an aqueous sample through a thin film of organic solvent, which is loaded as a supported liquid membrane (SLM) in the pores of a porous hollow fiber’s walls, and end up in an acceptor solution located inside the lumen of the hollow fiber (Figure 1).
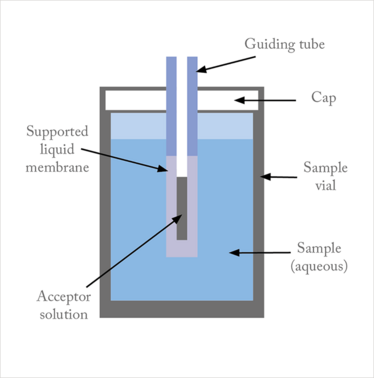
Figure 1. Principle of LPME
Three-phase LPME can be used for extraction of basic and acidic analytes, based on the fact that partition of such substances in and out of organic solvents is strongly pH dependent (Figure 2). Thus, for basic analytes, the sample is made alkaline, and the analytes are effectively extracted into the SLM in their uncharged state, followed by diffusion across the SLM and extraction into the acceptor solution. The acceptor solution is acidic, the analyte molecules become ionized, and they are prevented from re-entering the SLM. For extraction of acidic analytes, the pH-gradient across the SLM is reversed. After extraction, the acceptor solution is collected for the final analytical measurement.
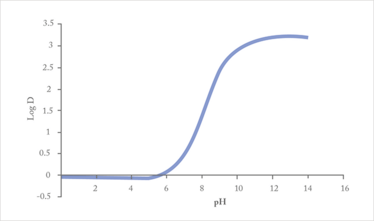
Figure 2. Partition coefficient (log D) for paroxetine between octanol and aqueous solution as function of pH. A high log D indicates strong partition into organic phase.
• Extraction into μL liquid volume directly compatible with liquid–phase separations techniques |
• No evaporation and reconstitution before chromatography/electrophoresis |
• Compatible with complex biological samples |
• Inexpensive and single-use |
• No carry-over |
• High pre-concentration |
The Solution
Sample preparation based on LPME has several advantages. First, LPME provides a high degree of sample clean-up from complex biological and environmental samples, because the non-polar nature of the SLM prevents most matrix components from entering the acceptor solution. For example, with human plasma samples, matrix components, such as proteins, salts, and phospholipids, are not extracted into the acceptor solution.
Second, LPME can provide high enrichment of analytes, due to the small (typically 5–25 µL) volume of the acceptor solution. From small biological fluid samples of around 250–500 µL, enrichment factors in the range of 10–20 are typically obtained. From large samples of environmental waters, enrichment factors exceeding 25,000 are possible (2).
Third, the acceptor solution is aqueous in the three-phase mode, and is directly compatible with liquid chromatography-mass spectrometry (LC-MS), high-performance liquid chromatography (HPLC), and capillary electrophoresis (CE), with no evaporation and reconstitution required. In two-phase LPME, neutral analytes can also be extracted, and because the acceptor solution is organic, two-phase LPME is directly compatible with capillary gas chromatography (GC).
We carried out the initial proof-of-principle experiments for LPME in 1998, and patented the work together with the university’s technology transfer office. We were inspired to a large extent by the development of solid-phase microextraction (SPME) (3) and SLM extraction (4). Personal contacts put us in touch with Varian, Inc (Torrance, CA, USA), who licensed the patent application. We worked with scientists at Varian to develop a prototype 96-well plate system for LPME (Figure 3) – a prototype that was tested in several US pharmaceutical industry laboratories, and performed well. However, Varian ultimately decided not to bring the 96-well LPME technology to the market.
The project with Varian was terminated for several reasons, including:
- Poor extraction kinetics due to inappropriate device geometry
- High estimated costs of mass production of the technology
- High estimated costs to develop an application database
Termination of the collaboration was a major disappointment. We later had discussions with a couple of other US-based companies about licensing the technology, but with no success. As university professors, we learned a lot from this industrial collaboration; the industrial mindset is very different from academia, and we now keep the industrial viewpoint in mind during our fundamental research. In particular, the experience has made us much more critical; we now aim for the technology we develop to be highly competitive in terms of workflow, costs, speed, and automation.
Beyond the Solution
Although LPME was invented more than 15 years ago, there is still significant interest in the technique within the scientific community, and around 80 research papers are published every year utilizing LPME. The majority of these papers are related to biomedical, environmental, and food and beverage applications. However, recent trends include the use of electrical fields across the SLM, and different hyphenations with molecular-imprinted polymers and nanoparticle technologies, to mention just a few.
In addition, several papers on automation have recently been published. Automation is mandatory for implementation of LPME in routine laboratories, but there is currently no commercially available equipment to allow this. Therefore, we recently developed a variant of LPME, termed parallel artificial liquid membrane extraction (PALME), which is based on existing 96-well plate technology intended for filtration (5).
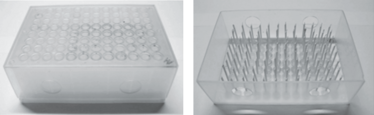
Figure 3. 96-well LPME equipment
We first published our work on PALME in 2013 (5), and filed a patent application in collaboration with the University of Oslo’s Technology Transfer Office. The principle of PALME is illustrated in Figure 4. The equipment comprises a 96-well sample plate and a 96-well filter plate. In the bottom of each well in the filter plate, there is a small filter of a porous polymer (100 µm thickness), and this serves as support for the SLM. First, the samples are pipetted into the sample plate. Second, 3–5 µL of organic solvent is pipetted into the filters, and the immobilized solvent serves as the SLM. Third, acceptor solution is pipetted into the reservoirs above the filters in the filter plate. Finally, the sample plate and filter plate are clamped, and agitated for 30–60 mins. During agitation, target analytes are extracted from the sample, into the SLM, and further into the acceptor solution.
Compared to LPME, PALME is restricted to relatively small sample volumes due to the 96-well geometry. The technology fits very well into pharmaceutical and biomedical laboratories, where sample volumes of 10–1000 µL are the norm. In contrast to LPME, PALME can be performed with industrial equipment – all liquid handling can be performed with multi-channel pipettes, and PALME has a high potential for automation. The advantages of LPME are maintained: no evaporation and reconstitution, excellent sample clean-up, moderate analyte enrichment, and only 3-5 µL solvent consumption per sample. The enrichment in PALME is less than in LPME, but sufficient for most pharmaceutical and biomedical application when combined with LC-MS.
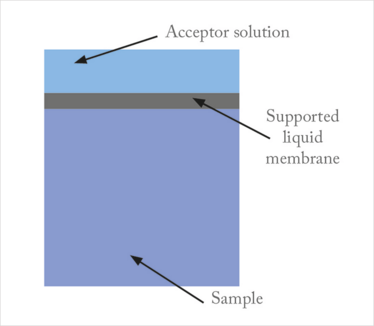
Figure 4. Equipment and principle
for PALME.
To date, our work with PALME has been performed with commercially available 96-well plates (5-8). The filter plate is the most critical part of the PALME equipment, and in our initial work we used filter plates equipped with polyvinylidene fluoride (PVDF) filters. However, this polymer is not ideal and we have observed non-specific binding of target analytes (5). Non-specific binding can be reduced or circumvented by careful optimization of the chemical composition of the SLM, using mixtures of organic solvents. However, this complicates PALME method development. Therefore, we are developing special 96-well filter plates optimized for PALME, which are not prone to non-specific binding. With this product available in the near future, setting up PALME methods in routine laboratories showuld be feasible.
Like all other methods, PALME has its advantages and its limitations. Based on current knowledge, PALME is ideal for extraction of basic and acidic analytes with a log P > 1 from aqueous samples, and for use in combination with LC-MS (and HPLC and CE). Such applications are common for drug analyses conducted by pharmaceutical companies, hospitals, analytical services contract laboratories, forensic toxicology laboratories, and doping laboratories. For final acceptance and implementation into routine use, the release of a commercial product optimized for PALME is critical (as discussed above). Longer term, development of extraction protocols for more polar analytes will also be an important step forward.
We welcome collaborators who wish to assess PALME – as well as the constructive comments and even criticism that follow. We would also be interested in talking with any industrial partners who would like to consider the potential of commercialization.
We are passionate about bringing the excellent performance and simple workflow of PALME into routine use, and we see our enthusiasm reflected in the eyes of our collaborators.
Stig Pedersen-Bjergaard is a professor in the Department of Pharmaceutical Chemistry, Astrid Gjelstad is an associate professor in the Department of Pharmaceutical Chemistry, and Knut Einar Rasmussen is professor emeritus – all at the University of Oslo, Norway.
- S Pedersen-Bjergaard, KE Rasmussen, Anal Chem, 71, 2650 (1999).
- TS Ho et al., Anal Chim Acta, 592, 220 (2007).
- CL Arthur, J Pawliszyn, Anal Chem, 62, 2145 (1990).
- S Palmarsdottir et al., Anal Chem, 69 1732 (1997).
- A Gjelstad et al., Bioanalysis, 5, 1377 (2013).
- M Roldán-Pijuán et al., Anal Bioanal Chem, 407, 2811 (2015).
- K Skoglund et al., J Pharm Biomed Anal, 129, 229 (2016).
- V Pilarova et al., J Chromatogr B, (in press).
Professor emeritus – all at the University of Oslo, Norway.