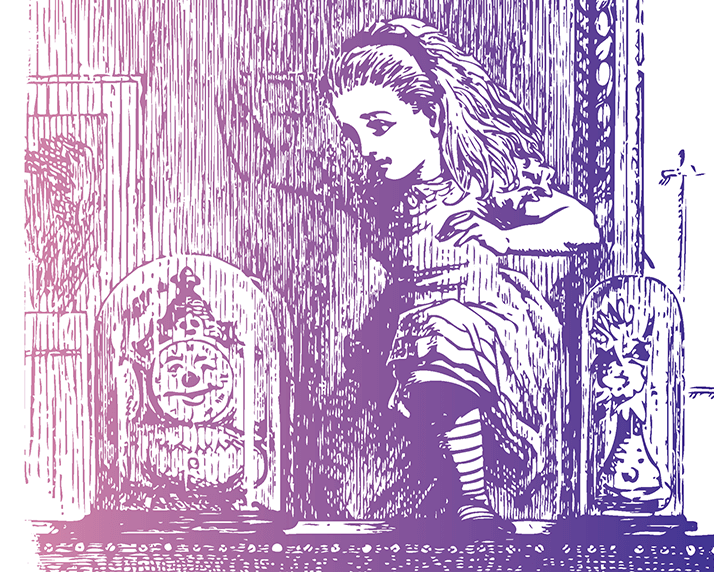
Christopher J. Welch
Is Science Lead for Analytical Chemistry within the Process and Analytical Chemistry area at Merck Research Laboratories in Rahway, New Jersey. He co-chairs the organization that oversees identification, acquisition and evaluation of new enabling technologies of potential value to Merck Research Laboratories. Welch has authored more than 200 scientific publications and patents and is co-founder of the journal, Enantiomer, and a member of the editorial advisory board for the Chirality, among others. Welch is also active elsewhere, including chair of the ACS Division of Organic Chemistry and a member of the Executive Committee for the International Symposia on Chirality. Welch’s father was an artist, grandfather a merchant and great-grandfather a preacher, and he feels that his career in the chemistry profession nicely combines these disciplines.
Yoshio Okamoto
Is a Professor Emeritus at Nagoya University, Japan. In 1979, Okamoto’s group obtained the first helix-sense-selective polymerization using triphenylmethyl methacrylate as a monomer and (–)-sparteine-n-BuLi complex as an initiator. The one-handed helical polymer (PTrMA) exhibited unexpected high chiral recognition ability. In 1982, PTrMA-coated silica gel was commercialized as the first synthetic polymer-based chiral column. In 1984, his group discovered excellent chiral recognition abilities with cellulose triphenylcarbamate and, in 1986, its 3,5-dimethyl derivative, which has been commercialized as Chiralcel OD. Okamoto’s group also reported amylose 3,5-dimethylphenylcarbamte (Chiralpak AD) in 1987. The latter two, OD and AD, are still some of the most popular chiral stationary phases used in HPLC and SFC.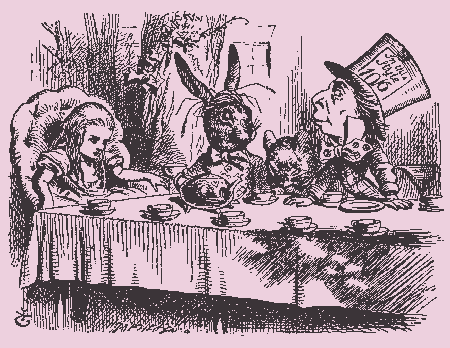
Bernard Testa
Studied pharmacy and undertook a PhD thesis on the physicochemistry of drug-macromolecule interactions and later attended to Chelsea College, University of London, as a postdoctoral research under the supervision of the late Arnold (Joe) H. Beckett. Testa’s fascination with chirality and stereochemistry began during his doctoral work and became a full-time pursuit under the supervision of Beckett, one of the very first scientists who grasped and explored the significance of stereoselectivity in drug and xenobiotic metabolism. Testa has held many prestigious academic roles, and (co)authored six books and over 500 research and review articles in the fields of drug design and drug metabolism. Testa’s Emeritus status has freed him from administrative duties and gives him more time for writing, editing and collaborating in research projects. Testa’s dedication to the field has continued ever since; “Organic Stereochemistry – Guiding Principles and Biomedicinal Relevance” (Wiley-VCH 2014) bears witness to his enthusiasm.Wolfgang Lindner
Is a Professor Emeritus at The Institute of Analytical Chemistry, University of Vienna, Austria. Wolfgang describes himself not just as an analytical chemist, but also a materials scientist, and he was trained as an organic chemist. Therefore, he attempts to bridge many different fields. Wolfgang is author of over 300 original publications, 11 book chapters, and the winner of numerous prestigious awards, including The Chirality Medal in 2008. In 2014, Wolfgang was co-chair of the International Symposium of Chromatography and he is active on several editorial advisory boards, including the Journal of Chromatography B and Chirality. Wolfgang co-invented chiral stationary phase technology that was licensed out to Chiral Technologies.Christopher Welch: Molecular chirality is very important in biomedicine. As the cellular machinery of life is asymmetric, the interaction of the two mirror image forms of chiral molecules can show different activities. Also, almost all new chiral drugs are prepared as pure enantiomers. Yoshio Okamoto: The homochirality of many functional proteins means that most biosystems (including human beings) are very sensitive to the chiral nature of compounds. Therefore, care must be taken when using biologically active chiral compounds. Chirality helps us to understand most stereochemical processes in biosystems. The distinct differences in the biological functions between the enantiomers of a chiral compound have long been understood, and the use of single enantiomers of chiral drugs was recommended about 30 years ago.
Wolfgang Lindner: I believe chirality and, therefore, the three-dimensional (3D) orientation of dissymmetrical small molecules in relation to large molecular objects – and them being surrounded by and in contact with chiral objects (molecules) – is intrinsically important. The diverse elements of molecular chirality describe a 3D information system on a macro molecular scale, which is essential for regulating stereochemically driven biological processes. Bernard Testa: The vast domain comprising the chemistry of stereoisomers includes all molecules that exist in two enantiomeric, chiral forms. However, stereochemistry extends well beyond chirality as it also includes an additional relevant property: diastereomerism. Many molecules have one or more diastereomers without being chiral, and there exists an even larger number of other molecules exhibiting both enantiomerism and diastereomerism (1). Stereoisomers are defined as molecules containing the same atoms (identical composition) and the same connectivity (identical constitution), yet they differ in their 3D-geometry (configuration). This implies that stereoisomeric molecules have an addition level of structural complexity compared to their non-stereoisomeric analogs. Therefore, I think it is better to focus on stereoisomerism rather than on the narrower field of chirality.
YO: Biot discovered optical activity in 1812 and Pasteur discovered the first resolution of tartaric acid in 1848, but the importance of chirality was better understood when in 1874 van’t Hoff proposed the tetrahedral arrangement of valence bonds in carbon. WL: Although Pasteur’s brilliant discovery of molecular chirality was more than 150 years ago (1848), it remains a hot topic encompassing diverse fields of natural, life, and material sciences, astrophysics, and even philosophy. CW: Pasteur’s, van’t Hoff’s and Kelvin’s contributions are well known, but it’s interesting to note that back in the late 1700s, Kant (Prolegomena to All Future Metaphysics, 1783) gave a very explicit definition of chiral objects. “What can more resemble my hand or my ear, and be in all points more like, than its image in the looking glass? And yet I cannot put such a hand as I see in the glass in the place of its original...”
But the history of chirality goes back even further. Aristotle, in De Incessu Animalium states, “All animals which are able of themselves to make a change of place, have a further distinction of left and right.” There are even earlier examples – although not so clearly stated. BT: A detailed and well-documented response to this question can be found in the September 2013 issue of Helv Chim Acta (5), which discusses pioneering work by Pasteur and Piutti. The latter of the two discovered D-asparagine and found that it tasted intensely sweet, in contrast to the tasteless L-asparagine, which laid the foundation for stereoselectivity.
CW: Biot’s discovery of optical activity and polarimetry was the starting point. For many years polarimetry remained the way to study and measure enantiopurity. YO: After Pasteur established several resolution methods that led to efficient separation of enantiomers, many groups developed an interest in analyzing chirality – but that was before chromatographic separation, the importance of which Wolfgang notes below. WL: The principle of discriminating enantiomers in a mixture goes back to Pasteur in 1861. After that it became the focus of diverse research groups worldwide, although it turned out to be a difficult task. Real breakthroughs, however, are associated with the development of separation sciences – in particular with capillary gas chromatography and liquid chromatography. These technological, scientific developments accompanied the steadily increasing knowledge of the principles of molecular recognition and intermolecular interactions for selectivity tuning. This new learning includes a better understanding of fundamental issues related to the inherent stereochemically controlled 3D structure of small molecules, and also of macromolecules and chemically modified surfaces.
For direct discrimination or resolution of enantiomers a chiral source (a chiral selector – SO) is required. The SO enables the enantiomers (analytes, selectands – SA) to have close contact with predominantly stereo-controlled multiple intermolecular interaction forces, which creates diastereomerically behaving SO-(R)-SA and SO-(S)-SA molecule associates that differ in their Gibbs binding energies. With the dominant liquid chromatographic enantiomer separation concepts we use today, the chiral selector is bound to a surface of a chromatographic support material called a chiral stationary phase (CSP). The difference between the free binding energies of the intermediate diastereomeric complexes is directly proportional to the observed enantioselectivity value α. BT: Interest in chirality grew with the availability of suitable analytical tools. These early chiral handles included chiral counterions, derivatizing agents, chromatography columns and chiral shift reagents.
CW: The origins of chiral chromatography are interesting and have strong connections with Tsvet’s development of chromatography in 1904. That year, Willstadter speculated about selective adsorption of chiral dye enantiomers by enantiopure silk or wool. It was a good idea, and attempts to actually do it were described in scientific literature for about 20 years, but there was no real progress with the technique. In the 1930s, Science reported on the separation of enantiomers of organometallic compounds using a column made from crushed single quartz crystals (quartz crystallizes in a chiral space group, and single quartz crystals are enantiopure). However, I think that when Prelog resolved the enantiomers of Troger’s base on a lactose column in 1944, the world saw the true start of chiral analysis. During the 1950s, Japanese researchers reported the separation of amino acid derivatives by paper chromatography, and in the 1960s, Gil-Av prepared several synthetic chiral stationary phases – that launched the modern era of the technique.
Baczuk et al. reported on the first chiral stationary phase designed for a particular molecule (DOPA) in 1968, and in the late 1970s, Cram described the development of a crown ether stationary phase with remarkably high enantioselectivity for amino acids. Throughout the 1970s and 1980s, my mentor at the University of Illinois, William Pirkle, designed and tested several chiral phases, which laid the foundation for future development of the field. I was an undergraduate in the Pirkle labs in 1981 – just at the time that the first chiral stationary phases were being commercialized – and I have been involved with this intriguing area of science ever since. Also, in the early 1980s, Yoshio Okamoto developed the polysaccharide based stationary phases that remain the most widely used columns today. Other important contributions in chiral stationary phase design during the 1980s came from Daniel Armstrong and Wolfgang Lindner, among others. YO: I think the first baseline separation of enantiomers using gas chromatography by Gil-Av in 1966 was an important breakthrough. Although it took many hours to complete the separation of enantiomers by capillary GC columns, it showed clearly that chiral analysis was possible by chromatography. Another important milestone was the first baseline separation of amino acids by Davankov in 1971 using liquid chromatography. It indicated the future possibility of efficient enantiomer separation by HPLC.
WL: Yoshio and Christopher have touched on the main names – I’ll provide a little more technical insight. In the 1960s, Davankov described the first fully designed and synthesized CSPs that enabled enantioselectivity for amino acids with intermediate diastereomeric metal mediated chelate complexes using proline derivatives as SO motifs. Then, in the early 1970s, Pirkle published an alternative concept of chiral discrimination based on intermolecular hydrogen bonding and π-π interactions between SO and SAs. In the late 1970s and early 1980s, the use of specifically modified (for example, polyacrylamides of amino acids and polysaccharides) chiral polymers as SOs and CSPs were discovered by Blaschke and Okamoto. Such polysaccharide type CSPs dominate the market today, as noted by Christopher. However, in the 1970s it was discovered that intrinsic stereochemically controlled 3D structures, such as proteins, could be considered as well as chiral polymers, and when bound to a support material they could be used successfully as CSPs.
These early developments – mainly driven by Hermansson and Allenmark – led to the commercialization of the first chiral columns offering a broad spectrum of enantioselective resolution for chiral drugs and intermediates. Those early pioneers enabled further SO types and principles to be described using various macrocycle type SOs introduced by Armstrong. In addition, the development of chiral ion exchangers is also significant to the success of CSPs and SOs. I also agree that credit should be given to the development of enantioselective capillary GC, pioneered in the late 1960s and early 1970s by Gil-Av, which was developed further by Schurig and König. The pioneering efforts made in enantioselective chromatography throughout the 1970s and 1980s and into the 21st century has enabled the technology to mature to a standard that allows us to resolve more than 90 percent of all chiral compounds (except carbohydrates) analytically – and also preparatively.
YO: Chiral analysis is the basis for developments in chirality. Most research and developments dealing with chiral compounds and materials use this efficient method. Without analysis, advances in chirality would be significantly retarded. For example, many papers have been published on asymmetric synthesis, and most of them used chiral analysis methods developed in the past three decades. CW: It’s very clear that the entire chirotechnology revolution in chemistry was enabled by the ability to keep score using chiral chromatography. This is now widely accepted, and one of the first masters of enantioselective synthesis, Meyers, was fond of making this point. I had the good fortune of being able to collaborate with him on some early alkaloid enantioseparations during my undergraduate days in the Pirkle lab. WL: For all the diverse chiral technologies in use and being developed by industry, academia, and government laboratories, the availability of stereoselective and quantitative analysis methods and tools are essential. Breakthrough contributions into the field of chromatographic sciences have established the basis for the high technical standards we have today. There are so many contributors and we should be grateful to them all for getting us to the level we are at today. BT: I’ll conclude this point on a higher level: basic research depends on better and new technological tools as much as technology needs fundamental discoveries to progress. Applied and basic sciences therefore complement each other in a hopefully never-ending spiral of progress.
BT: One important trend that springs to mind is the constant improvement in computational resources for maximizing response-to-noise ratios. YO: Development of new stationary phases is still continuing both on molecular-type and polymer-type stationary phases. The sensitivity of chiral detectors is still not sufficient; better detection of chirality is highly expected and miniaturization is a recent trend in separation science. WL: Although we are achieving a high standard of chromatographic resolution of enantiomers, further advancements will continue. Such advancements are chemically driven in terms of the search for new chiral selectors (CSPs) but also technologically driven in terms of the implementation of materials with higher efficiency and higher loadability. CW: Speed is important. Many chiral chromatographic separations are possible in less than a minute, and we have recently shown that a number of separations are achievable in seconds. This is very important for high throughput analysis, where hundreds or thousands of samples must be analysed in a single day. In addition, there is continuous progress in increasingly efficient chiral columns – the sub 2-micron columns combined with the new Waters UPC2 instrument are pretty amazing. Finally, there is an ongoing quest for additional universal stationary phases – columns that will work well for many different analyte structures.
CW: We have some excellent work progressing in our labs, and there are a lot of good things appearing from both academic and industrial labs worldwide. For example, we are seeing some important work on chiral sensors – either optical or MS-based. The key problem of interference with matrix components is beginning to be addressed, and these techniques show some pretty good promise. In particular, the work of Anslyn (University of Texas) and Wolf (Georgetown University) stands out. YO: I think Armstrong (University of Texas) and Lindner (University of Vienna) are excellent examples. Also, companies such as Chiral Technologies and Phenomenex are helping advance research. Within China, this area is rapidly advancing too. WL: I find it difficult to answer the question objectively. However, the most advanced chiral columns are provided by Chiral Technology Europe, Phenomenex, Macherey & Nagel, Sigma-Aldrich, Regis, AkzoNobel, and so on.
CW: I think the importance of chirality and chiral analysis is pretty well understood nowadays. I remember the strange looks I used to get in the early 1980s when I tried to share my research interests with others; now, high school students are learning about enantiomers, racemates and resolutions. YO: The importance of chirality is understood sufficiently within the chemical and pharmaceutical industries. However, it is not fully appreciated by other industries and by governments. But I must say the situation has improved since the Nobel Prize award to Sharpless, Noyori and Knowles for asymmetric catalysis in 2001. BT: Definitely not. There is a general ignorance in official and medical circles of what stereochemistry is, does, and contributes to scientific understanding. We need to adopt a common language when communicating about chiral analysis and its benefits to others; such a common language already exists within chemistry, stereochemistry and chirality (6). Some biomedical workers ignore or misuse stereochemical nomenclature in their publications. For example, by using ambiguous lower case d and l, either for optical rotation or for fake descriptors of absolute configuration. Some authors may be at a disadvantage due to a lack of instruction at pre- or postgraduate levels, plus there may be a lack of thorough in-house training within their place of research or work. And what about copy editors whose expertise or allocated time is wanting? Also optical purity does not receive the attention it deserves, considering how much it may affect pharmacological outcomes (1).
CW: A bold prediction: how about handheld device offering same day method development for wide variety of compound structures, delivering a <15 s assay time for all but the most difficult separations? But maybe that will take 11 years... YO: Success rates for chiral analysis (separation) are already high, and many chiral compounds can be analyzed by GC and HPLC using various CSPs. However, the chiral recognition mechanism of these phases, particularly polymer-based phases, is not obvious. This situation will be improved over the years; finding the solution may lead to the design and synthesis of new chiral phases. From an environmental improvement viewpoint, separation by supercritical fluid chromatography will become more important and popular. WL: Progress in chiral analysis methodology will involve separation sciences, spectroscopy, physics, and also, more broadly, chemistry, material sciences and biochemistry. Chirality related research is, therefore, interdisciplinary, and analytical sciences provide essential tools to tackle the manifold challenges. Although we have achieved high technological standards, the prediction of enantioselectivity and resolution values for certain chiral selectors and CSPs for resolving some chiral analytes remains largely trial and error. So, the full understanding of the underlying mechanisms and dynamics of molecular recognition and stereoselective discrimination processes remains challenging and is an extremely exciting field of ongoing and future research. As a driving element that plays a central role in chemistry, biology, and material sciences, chirality requires continuous research to further advance its impact. The tools and methodologies required to analyze chirality, to discriminate between stereoisomers, and to resolve the enantiomers of a mixture, are indispensable in moving chirality-driven science forward.
By Wolfgang Lindner The last 30 to 40 years of enantioselective chromatography is a true success story. Unfortunately, it was essentially driven by the pharmaceutical industry’s Thalidomide (Contergan) tragedy in the late 1950s and early 1960s... The commercial availability of these chiral columns stimulated research on the pharmacological difference of chiral drugs leading to modern guidelines that each stereoisomer of a drug
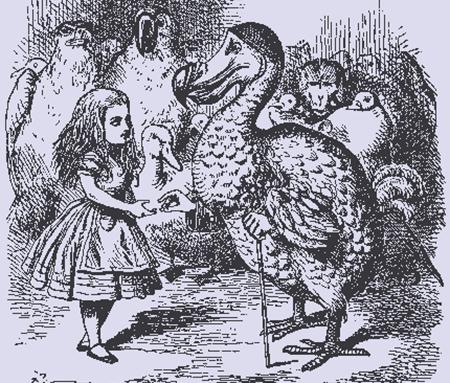
substance with chiral centers needs to be produced as stereochemically integer drug substances for pharmacological testing. These stereoisomers also need analyzing stereoselectively in terms of enantiomeric purity. The success of the pharmaceutical industry also inspired the food and agrochemical industries, together with those involved in pure and applied chemistry, to investigate the new analytical possibilities of chiral analysis. As robust enantioselective separation methodologies improved rapidly, they became an indispensable method for the characterization and analysis of any small molecule type chiral compound that was newly synthesized or was becoming part of a multi-step synthesis protocol. This relates to the extremely dynamic field of asymmetric catalysis for organic, pharmaceutical, and agrochemical chemistry, and the further progress of reaction performance should be monitored and analysed. Preparative chromatography technology for resolving enantiomers from a racemic mixture has also improved significantly. In this context, the establishment of techniques such as simulated moving bed (SMB) chromatography needs mentioning; this has become the method of choice for the chemical and pharmaceutical industries for isolating chiral compounds on a multiton scale for resolving enantiomeric species. We should also mention technological advancements of super critical fluid (or super-fluid) chromatography (SFC) for (semi)prep chiral resolution from the mg to the kg or even higher level. Economically, enantioselective SFC is the first choice for such resolutions.
By Bernard Testa The big question: is chirality (specifically) and stereochemistry (more generally) essential for the emergence and evolution of chemically based life? We still seek the answer, but do know that the additional level of complexity caused by stereoisomerism also implies additional/emergent properties (2).
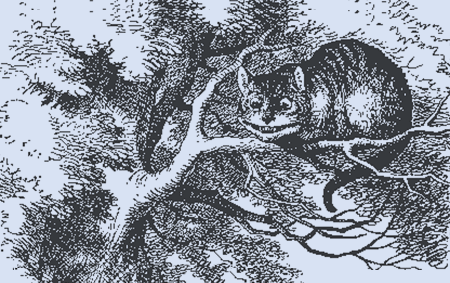
My answer is: stereoisomers contain additional and highly valuable information compared with their non-stereoisomeric analogs. The supplementary information provides a broader range of responses (outcomes) in the interactions and reactions of stereoisomers, suggesting that stereoisomerism indeed contributes – perhaps critically – to the chemical complexity underlying life. The operational concept here is stereoselectivity, namely the differential interactions of stereoisomers with enzymes, transporters, receptors, and other functional macromolecules. Such stereoisomers can be endogenous compounds (such as hormones and neurotransmitters) or indispensable exogenous compounds (for example, nutrients and vitamins), and these usually exist in a single stereoisomeric form progressively selected by evolution to support life from among a mixture of stereoisomers. Casual exogenous compounds are known as xenobiotics and include drugs, recreational compounds, natural toxins, and pollutants. When present as stereoisomeric mixtures, xenobiotics interacting with biosystems may elicit an overall response that results from the combination of two or more distinct outcomes.
The above scenarios outline the concept of substrate stereoselectivity. But there is more to the story, since the enzyme-catalyzed metabolism (or biotransformation) of endogenous and exogenous substrates may generate new stereogenic elements in a metabolite. For example, a center or an axis of chirality, or (E,Z)-diastereomerism. Such a reaction needs prostereoisomerism in the subtrate molecule, for example, a prochiral center. In most cases, the stereoisomeric metabolites are not generated at identical levels, a phenomenon that gave rise to the concept of product stereoselectivity (3), which was developed further by Prelog (4). Since the chemistry of life is consistently more complex than we expect, product stereoselectivity may also differ between stereoisomeric substrates, a phenomenon known as substrate-product stereoselectivity. Therefore, the additional structural information contained in stereoisomeric molecules may elicit an increased number of outcomes compared with non-stereoisomeric molecules. Stereoisomeric molecules allow more information to be gained from their interactions with biosystems, which themselves are endowed with chirality and stereoisomerism.
References
- B. Testa, J. Caldwell, M. V. Kisakürek, Eds. “Organic Stereochemistry Guiding Principles and BioMedicinal Relevance”, (Wiley-VCH, Weinheim, Germany, 2014). B. Testa, G. Vistoli, and A. Pedretti, “Small Molecules as Exemplars of Emergent Properties and Diversification into the Adjacent Possible”, Chem. Biodivers., 11, 1309-1329 (2014). B. Testa, “Types of Stereoselectivity in Drug Metabolism: a Heuristic Approach”, Drug Metab. Rev. (2014) DOI: 10.3109/03602532.2014.984814 www.nobelprize.org/nobel_prizes/chemistry/laureates/1975/prelog-facts.html J. Gall, “Molecular Chirality in Chemistry and Biology: Historical Milestones”, Helv. Chim. Acta., 96, 1617–1657 (2013). IUPAC (International Union of Pure and Applied Chemistry), “Recommendations 1996 - Basic Terminology of Stereochemistry”, Pure Appl. Chem., 68, 2193-2222 (1996).