I have been interested in both science and history since childhood, and though I ended up specializing in science, I remained fascinated by the past. During the final year of my integrated chemistry degree at Oxford University, I was offered a one-off opportunity to work in an archaeology research lab, studying nitrogen isotopes to learn about the diet of Paleolithic humans. Within weeks, I knew it was exactly the type of research I wanted to do; being able to use chemistry to understand our past was a dream come true. I went on to a PhD project that focused on amino acid racemization (also known as amino acid dating) in fossilized shells at Newcastle University. I have been working in amino acid racemization of fossilized materials ever since.
A biological clock
My PhD supervisor, Matthew Collins, had a strong focus on archaeological science, with one set of researchers working predominantly on bone and another on pottery, but I was the only one working on shells and focusing on their potential for dating. After a fantastic three months being trained at Northern Arizona University with Darrell Kaufman, I set up the amino acid lab in Newcastle. Towards the end of my PhD, the lab moved to the University of York, and is now the NERC-recognized lab for amino acid analysis - North East Amino Acid Racemization (NEaar; http://tas.txp.to/1117/NEaar). Anybody can analyze a fossil but, when it comes to geochemistry, the key issue is: how do we really know if we are looking at the original molecules? We’re dealing with incredibly low concentrations and a high risk of contamination. I’m interested in amino acids, but the sample will also contain minerals, fats and sugars, and all sorts of other things that can potentially compromise or complicate the analysis. The tricky bit is being able to isolate the part you want to look at, without altering it in the process. Working at low concentrations always pushes the boundaries of our methods and limits of detection – it’s analytically very challenging, but that’s what makes it so rewarding!The core goal of my PhD was to isolate a fraction of protein from fossil shells that wasn’t in contact with the environment, but locked away within the mineral lattice of the shell – effectively acting as a closed system. The reactions that the protein is subjected to in this intra-crystalline fraction are predictable, making it possible to use these to accurately date the sample. To isolate such preserved proteins, we pre-treat our samples with bleach, to remove contamination and any exposed (open system) amino acids (1). We also routinely analyze multiple amino acids – both free amino acids (FAA) and total hydrolysable amino acids (THAA) – as these show different levels of protein breakdown that are highly correlated (2). It is this combination of bleach pre-treatment and analysis of multiple chiral amino acid analysis that allows us to measure the “Intra-crystalline Protein Decomposition” (IcPD), which is affected only by time and temperature. In this way, we can draw conclusions about the age of the sample and the temperatures it has experienced. I soon found that some minerals hold onto this intra-crystalline protein much better than others. During my postdoctoral research, I started to look at the stability of the minerals themselves, to understand why some were more “closed” than others – and that’s when I started focusing on calcitic material. Calcium carbonate exists predominantly in two forms within biominerals: calcite and aragonite. Aragonite is the main component of shells but is less stable than calcite over geological timescales. Looking at the way aragonite breaks down helped us understand that calcite is a much better biomineral to target for longer timescales (3). We published work last year (4) on the isolation of peptide sequences from four-million-year-old eggshells; the stability of the calcite meant that the peptides incorporated within the intra-crystalline fraction stayed integral to the fossil material.
Opening a door to the past
Luckily, the opercula – the “trapdoors” snails use to shut themselves away inside their shells – of one abundant fossil species are made of calcite, and since 2005 I have been dating these calcite fossils at a whole range of sites across Europe. The dating method covers the Quaternary Period (the last 2.5 million years), which is a very difficult period of time to date, but very important; understanding climate change in the past is essential when projecting the potential effect of our future climate. During the Quaternary Period, the climate of the Earth has moved between warm conditions similar to today, to having ice sheets as far south as London. We’ve been dating a whole range of archaeological and geological sites over that period, and that’s helping us build up a really interesting picture, both of our human past and of the Earth’s climate over geological timeframes. We work at sites containing the earliest evidence of human occupation in Europe, so we can start to see under what conditions these humans were living in and how that relates to the climate at the time – plus we have gathered some very interesting climatological data at a whole range of other sites. At one particular site in Germany, amino acid dating and other dating methods (including measuring the “flip” of the magnetic poles) showed that, during the last warm stage (~125,000 years ago), central Germany started warming as much as 2,000–5,000 years after Greenland. Such a large delay was incredible and unexpected, as it was thought that global temperature increases would be reflected locally much more quickly. This has implications for how we understand the way the Earth’s system worked; for example, the impact of feedback effects, when the Earth’s response to a climate driver can positively or negatively amplify the original change. We’re still investigating how we can integrate that type of study into current climate models. The opercula dating work was really painstaking – analyzing the protein in nearly 1,000 tiny sesame seed-sized fossils is no mean feat. But it’s been more than worth it to open a window into the climate over the last two million years, as well as on our human evolutionary story.Teaching the next generation of geochemists Teaching is an integral part of my role at York. As well as supervising PhD students, I teach undergraduate courses in separation science and on geochemistry, which look at what chemistry can tell us about the past climate of the earth, how it’s used in archaeological science to help us understand our human past, and so on. To succeed in analytical science, you need the desire to learn more and the desire to push the boundaries. The yearning to do something different (and be happy to work hard at it) and the ‘gleam in your eye’ when you talk about an unanswered question – I think they are essential elements of an analytical career. Many of my research group members have very different ways of working to me, but they all have the same drive. I try to foster that spirit of discovery in my students, both undergraduate and graduate. I even use The Analytical Scientist in my teaching because it covers a broad range of different research questions and applications; several of those case studies have been used in my courses. I love chromatography, but even I must admit that teaching somebody about plate heights and the van Deemter equation can be a little bit dry. Being able to point to a really interesting case study shows students that all the theory can actually make their separations better – and allow them to do things that other people can’t. One slightly different assessment method I use on my geochemistry course has proved really popular. I get the students to work in groups to design a small wiki-based website on a lecture topic that they have delved deeper into. The interdisciplinary nature of the subject lends itself well to a diverse range of topics, and I’ve been really intrigued by the areas that they’ve chosen. The students then evaluate each other’s websites; I’ve been so impressed by the care and attention they put into that feedback – really thoughtful, insightful, positive comments. I’ve never had to edit them. Peer-to-peer evaluation is such a powerful method of getting students to think about what works and what doesn’t. It’s about communication: making sure you get the science right, but also making it accessible, interesting and engaging for your audience – that’s a hugely valuable skill in any realm of science or business, and it is wonderful to see our students rise to the challenge.
Working knowledge
For me, analytical science has always been a collaborative discipline, and choosing particular projects is often serendipitous. You might have a conversation with another researcher, and hit on an interesting problem, or your research might take you off in an unexpected direction. I’ve never really sat down and planned what I’ll be working on in a year’s time – instead, projects often come out of talking to other scientists and realizing that you might have the tools or background knowledge to tackle a problem that is otherwise unanswerable. For example, we’re looking at very long-lived corals in a new project. Originally, we wanted to see if we could date them – and we could – but then our interest was piqued by the perturbations we saw in some of the protein data; it looked as if the corals were laying down slightly different proteins, possibly in response to environmental stress. I’m now working with Bristol University and St Andrew’s on two different projects to examine the way corals mineralize and how they respond to environmental stresses, such as bleaching events or increased carbon dioxide concentrations. The interdisciplinary nature of my work means that I need to present our work to a broad range of audiences. I give talks to chemists and discuss the technical details of chromatography, but I also speak to archaeologists who’ve never given more than a fleeting thought to proteins. In my research group, we try really hard to tailor our communications in the right way.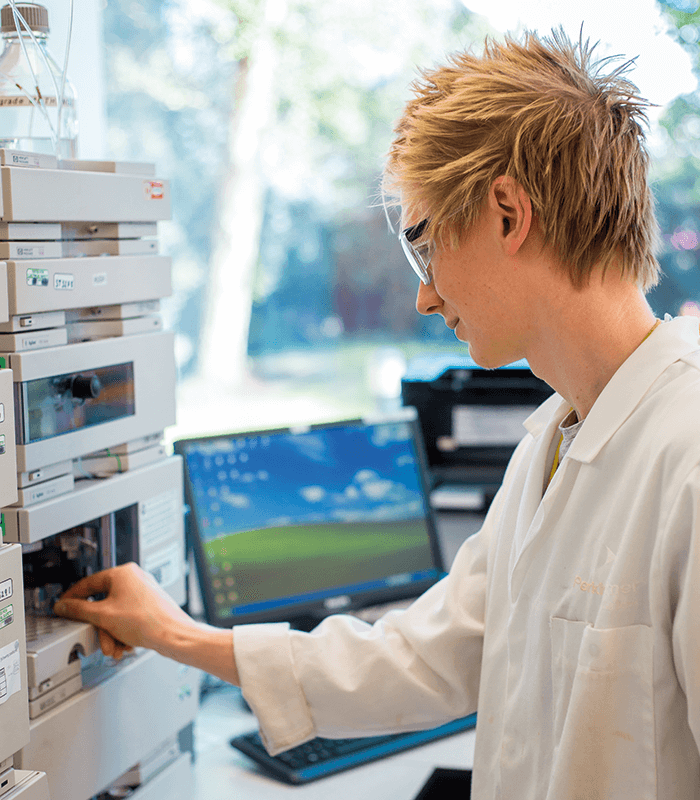
The only constant is change
I find my work incredibly rewarding. On a day-to-day basis, I love the tinkering, the testing, the optimization of methods and instruments – all the while knowing that it has an ultimate purpose, which makes it even more worthwhile. I find it very exciting to date sites that hitherto have been almost impossible to date – and then to relate those sites to either our understanding of our human evolution, or our understanding of the way our earth responds to its climate. Analytical science has a huge role to play in the future of geochemistry. Developments in analytical technology mean we can answer far more wide-ranging questions than we could 20, or even 10 years ago. With geological and archaeological samples, we’re working at trace concentrations in incredibly complex samples that have undergone fascinating breakdown reactions. It’s a big analytical challenge – and I think that’s why many of us are drawn to this type of application. Of course, Earth scientists are now becoming increasingly trained in the use of routine geochemical and archaeological science methods, so it’s not always necessary to have chemists performing these analyses. However I think there’s always a role for analytical chemists to take the science further, to develop better methods and improve technology, which can later be used by a whole suite of different scientists. As an academic researcher, I’m fortunate enough to have relative freedom to pursue my own research; as in most fields, it’s the funding that’s the issue. However, we’ve been relatively lucky in gaining funding for projects from a range of bodies because we’re working across different areas. At the moment, the research areas I’m naturally attracted to (for example, climate) seem to fit within the realms of what is considered useful. As funding gets more competitive and more government-targeted, it’s important to communicate effectively with funding agencies. The research question is key; if it isn’t tangible and easy to understand, then it is a struggle to get funding. You have to be able to articulate why it is interesting, important or has wider relevance. A fantastic research group and close collaborations with earth scientists and archaeologists have been integral to our research, helping us to push the analytical science forward, whilst advancing our understanding of our earth’s history. Being able to look at what’s happened in the past, and looking at the rates of change that we see, is really helpful for us in understanding – and therefore potentially mitigating – the impact of future change. The overwhelming majority of scientists who are working in this area believe that global warming is caused by human activities. It’s crucial to understand what the implications are, on both global and local scales; what is the impact on sea level, on weather patterns, on the environment, the flora and fauna? One problem is that I never really know what to call myself. I am an analytical chemist, but also a geochemist; my research also delves into earth science and oceanography; I look at biominerals, so materials science is also involved; and obviously there’s a good amount of archaeological science as well. I guess wearing all those different hats is what makes my work so exciting! Kirsty Penkman is a Reader in Analytical Chemistry, University of York, UK.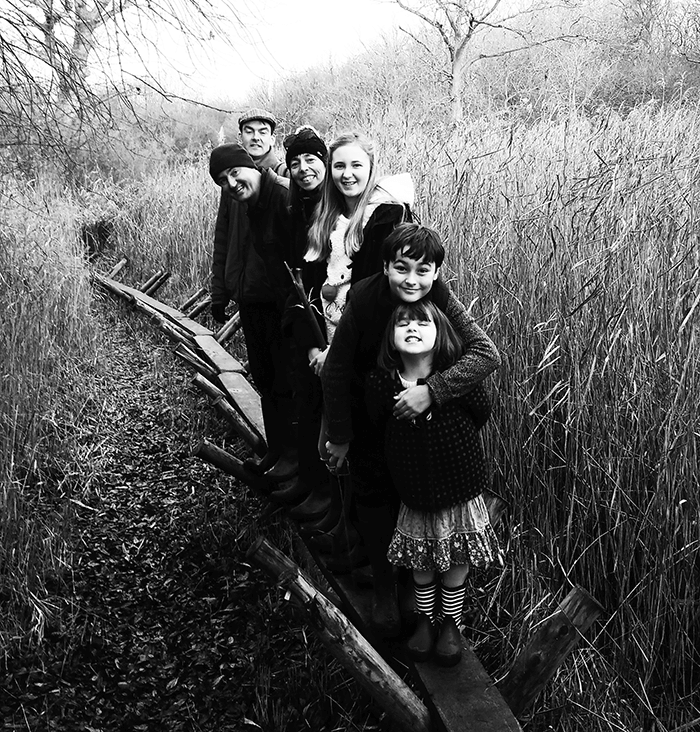
The Star Carr site was first discovered in the 1950s, and dates from 11,000 years ago – at the end of the last Ice Age, when Britain began to be repopulated by Mesolithic tribes. Because it was a wetland deposit, and the soil is peaty, there was phenomenal preservation of organic material, such as bone and wood, which isn’t preserved in the majority of archaeological sites of that age. It provided a unique perspective into the organic materials these hunter-gatherer populations made use of; archaeologists found the very first evidence of carpentry and about 20 headdresses made from deer antlers, making Star Carr a site of world importance. Work in the 1980s indicated a small amount of degradation in the organic material compared to the 1950s excavations. But when the site was re-excavated in 2007, the level of organic degradation in the same layers was horrifying. In the 1950s, they removed large numbers of bone, antler and wood fragments; in the 2007 excavation, only two very small bone pieces were found – and they were “sloppy,” having been demineralized in some way. And the wood’s cellulose had been stripped, leaving just the lignin skeleton. The York Archaeological Trust (YAT) looked at the wood, and we did the analyses to prove the bone was heavily demineralized. In late 2008, English Heritage, the Vale of Pickering Research Trust and a range of other stakeholders met to discuss what we should do about it, and we presented our findings on the bone and wood samples. However, although the state of the material appeared different to what had been excavated previously, there was no scientific data to answer some important questions. How rapidly had the degradation occurred? How stable was the remaining material? Without that data, English Heritage found itself in a very difficult position in terms of managing the site – should the site be left there or dug up? We decided to put together a NERC-funded CASE PhD studentship with YAT to answer some of these questions, and recruited a talented student: Kirsty High. We wanted to be able to look at a range of breakdown parameters: a range of different molecules, using techniques that were relatively inexpensive and widely accessible (e.g. LC, GC, XRD, FTIR), so that other people would be able to do the same work. We were able to show that the bone fragments were degrading rapidly and were very unstable under particular site conditions (5). Degradation of organic material was occurring within a year – we never expected to see so much breakdown in such a short time period. The message for those charged with caring for our archaeological heritage is that it can be really important to act quickly – and in the right way.
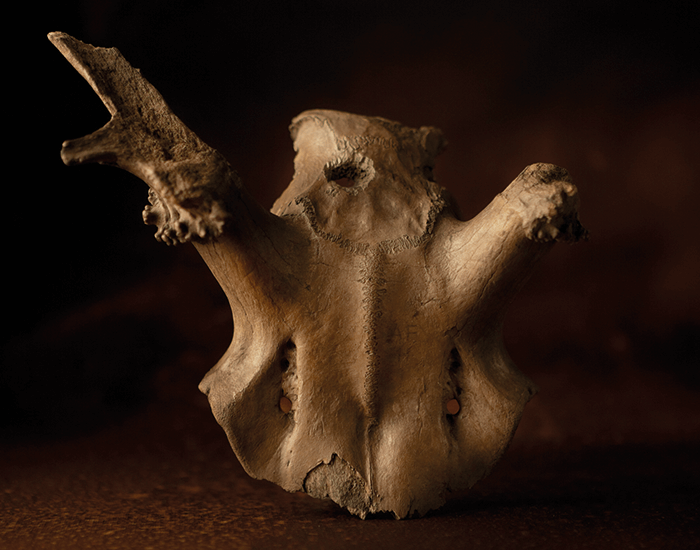
Kirsty now has a NERC fellowship working with Historic England (formerly English Heritage), which will enable us to use the data we generated to help protect other archaeological sites and ensure the best management decisions are made. Wetland areas like Star Carr are widespread across the world, and our work has shown that it’s critical to understand and monitor the conditions within these sites. If you see particular changes in environmental parameters, the assumption that material will be preserved “in situ” may no longer be valid. There’s a big move in archeology towards preservation in situ, so that artifacts remain available for better scientific techniques in future – but if you don’t monitor the sites properly, then they might not be there in the future. That’s what we’re seeing at Star Carr.
References
- KEH Penkman et al., “Closed-system behavior of the intra-crystalline fraction of amino acids in mollusc shells”, Quaternary Geochronology, 3, 2–25 (2008). RC Preece, KEH Penkman, “New faunal analyses and amino acid dating of the Lower Palaeolithic site at East Farm, Barnham, Suffolk”, Proc Geol Assoc 116, 363–377 (2005). KEH Penkman et al., “A chronological framework for the British Quaternary based on Bithynia opercula”, Nature, 476, 446–449 (2011). B Demarchi et al., “Protein sequences bound to mineral surfaces persist into deep time”, eLife, 5:e17092 (2016). K High et al., “Lessons from Star Carr on the vulnerability of organic archaeological remains to environmental change”, PNAS, 113, 12957–12962 (2016).