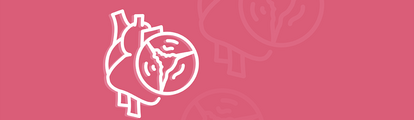
See the Light: Exciting Applications of Microscopy and Spectroscopy
When spectroscopy meets microscopy: from the origins of Stonehenge, to microplastic analysis, to protein localization in the aortic valve
When curating news stories for our fortnightly spectroscopy newsletter, we’re often struck by microscopy’s key role. Indeed, the two techniques are increasingly entwined. And that’s why we’re pleased to present in this bespoke newsletter, sponsored by Hamamatsu, a collection of research stories where spectroscopy and microscopy both play a crucial part.
Given the closeness of these two techniques, it would seem remiss of us to overlook microspectroscopy, which combines the fundamentals of both – enabling the study of microscopic materials through light–matter interactions.
Manufacturers are continually making advances in microspectroscopy, enabling novel research. Some of these advances are small incremental improvements (better optics or lasers, for instance), but there have been other more revolutionary developments in the pairing of microscopy with spectroscopy.
According to Brooke Kammrath and Dale Purcell: “Recent advancements in dichroic mirrors have enabled high throughput spectral analysis and confocality when using different modalities to analyze the same sample. Another trend is the pairing of different techniques with Raman microspectroscopy into instruments capable of simultaneous or tandem analysis on the same sample.”
Today, microspectroscopy is more than just a concept. “It is already established in forensic science and it is ready to expand to other fields,” they say.
Below, in addition to the microscopy and spectroscopy double act, we also shine a light on some recent applications of microspectroscopy that caught our eye – from microplastic detection to battery analysis.
The Analytical Scientist Presents:
If you're enjoying this article, you may like to join our mailing list to receive new content every fortnight. The Spectroscopy Newsletter offers the hottest topics at your fingertips, specially chosen by our wonderful Editorial team!
Foreign (Blue)Stones
A quick internet search about Stonehenge raises more questions than answers about the origin and history of the Neolithic monument. With the power of microscopy and spectroscopy, researchers from Italy, Canada, and the UK analyzed the mineralogy of the Stonehenge Altar Stone, revealing that its origin is not the same as the rest of the “bluestones.” This crucial detail could reveal more about the migration of the British Neolithic ancestors, their cultural and religious practices, and thus Stonehenge’s history.
“When Raman spectroscopy is combined with reflected light microscopy, [...] it is possible to identify the varieties of iron oxides/hydroxides and titanium oxides present and to separate one sandstone from another. This approach was fundamental in our study and enabled us to distinguish between samples of the Old Red Sandstone sequences in Wales. Such a high-resolution approach is required to fingerprint the possible source of rocks used in archaeological monuments.”
Life Found in Two-Billion-Year-Old Rocks
Living microbial communities have been discovered inside two-billion-year-old rock from the Bushveld Igneous Complex (BIC) in South Africa – the oldest finding of microbes in ancient rock to date. The research team employed a combination of infrared spectroscopy, electron microscopy, and fluorescent microscopy to confirm microbial cells densely packed into fractures, sealed off from the external environment by clay minerals.
An Automated Avenue for Microplastic Detection
An AI-based microspectroscopy imaging identification system, PlasticNet, can detect microplastics with high accuracy – and could be used in wastewater treatment and food production plants. The tool, developed by researchers at University of Waterloo, Canada, uses a deep learning convolutional neural network architecture to automatically detect images generated by focal plane array (FPA)-based micro-fourier transform infrared (FT-IR) microscopy.
After training PlasticNet with over 8,000 spectra of virgin plastic, and then retraining the tool using non-virgin plastics, PlasticNet was able to successfully classify 11 types of common plastics with 95 percent accuracy. The team also demonstrated a 17.3 percent improvement in identifying polypropylene microplastics compared with other environmental datasets.
“I was surprised by the efficiency of deep learning in this context,” says lead author Ziang Zhu. “With PlasticNet, we received precise and reliable results in a field that is known for often dealing with complex and variable data. It’s really opened up avenues for future investigations in environmental science.”
Also in the News…
Studies employing cryogenic electron microscopy (cryo-EM) have previously failed to fully characterize TMEM16F – a membrane protein involved in many physiological processes and COVID-19 pathogenesis. An international research team successfully identified its native structure and function using a combination of single-molecule force spectroscopy (SMFS) and high-speed atomic force microscopy (HS-AFM) imaging. Their work demonstrated the structure, dynamics, and mechanical properties of the protein – results that contradict the current theory that TMEM16F functions as a simple cell gate.
Researchers from Texas A&M University and the University of Notre Dame collaborated to examine plastic nanoparticles in water samples obtained from two open oceans with shrinking surface bubble deposition (SSBD). The technique was originally developed for DNA analysis combining electron microscopy and surface-enhanced Raman spectroscopy and repurposed for this study to enable nanoplastic size and morphology determination – a measure that current techniques, such as gas chromatography and mass spectroscopy, cannot provide. The team discovered nanoplastics made of nylon, polystyrene, and polyethylene terephthalate.
Faidra Amargianou and colleagues developed a multimodal X-ray microspectroscopy tool to characterize chemical interactions within battery materials at the nanoscale. The researchers believe their approach can also be applied to other laminated materials, such as graphene and thin film solar cells, “thereby opening new insights into intercalation and surface redox processes,” they concluded.
Researchers used optical microscopy, electron microscopy, and spectroscopy to investigate mineralization and protein localization in the aortic valve for the first time, finding that calcium phosphate in the mineral deposits is not the same type as found in bone. “These types GeoBioMed analytical approaches and experimentation will permit discovery of fundamentally new approaches for the development of clinical therapies targeting the prevention and treatment of aortic valve leaflet calcification,” the authors concluded.