The Six Dimensions of GC
The juxtaposition of simplicity through new technology with complexity in the chemistry keeps gas chromatographic method development exciting – despite GC being described by many as a “mature” technique. But are we still using packed-column thinking?
Can we argue that today’s analytical methods are both simpler and more complex than a generation ago – for example, when I did my first injection into a gas chromatograph? In 1985, many typical gas chromatographic methods employed a packed column with direct injection and detection by flame ionization or thermal conductivity detection. Although capillary columns had been in use for about 30 years, easy-to-use fused silica capillary columns had only been known for about five. Back then, most instruments for capillary GC were modified versions of their packed column cousins. The useful separation space on a typical packed column accommodated about 10 peaks, so chemistry optimization focused almost entirely on the column. There were hundreds of stationary phases available for packed columns, each with its own selectivity. Sample preparation chemistry was critical because interferences that would generate overlapping peaks or damage the column needed eliminating – therefore, packed column methods usually considered these two of the possible separation dimensions carefully.
GC’s roots in packed columns still influences us today; I call this “packed-column thinking”.
The practical consequences of the fundamental equilibrium theory driving separations has shifted. Due to the high mass of stationary phase present in packed columns, separations are performed at temperatures generally well above the normal boiling points of the analytes (thick stationary phases make analytes “stick” more strongly in the column). While in capillary columns, separations are usually performed at temperatures well below the normal boiling point (thin films favor evaporation into the mobile phase). This, together with engineering advances that easily allow hundreds of thousands of theoretical plates in capillary columns, has caused the range of possible analytes for GC to rise considerably.
Inertness was often a problem in packed columns. Indeed, the interior of a modern capillary GC column can be one of the most inert places in the entire field of chemistry. The column is manufactured from deactivated fused silica that is chemically bonded to a stationary phase usually made from a very high purity polydimethylsiloxane (silicone)-based polymer. And, the carrier gas is usually high purity helium. Simply put, if the inlet is kept clean, there is little available for labile compounds to react with, as long as air and water are kept out.
Today’s methods employing comprehensive two-dimensional GC (GC×GC) have up to six (yes, six – can you name them?) possible dimensions in which separation can happen, or in which separation can be compromised. Most articles and reports still focus primarily on optimizing one or two dimensions – rarely all six, just like the packed column literature of yesteryear. Papers and methods that focus on the columns rarely focus on the injection (in fact, relatively few papers seriously discuss optimization of the injection), sample preparation or detection. Papers that focus on sensitive detection, such as with mass spectrometry (MS) often treat the chromatography and sample preparation as merely an inlet. We are all guilty of this – a full optimization and discussion of all steps in the process could turn the average paper into a dissertation.
Here are some of the common statements about gas chromatography that, given the separation power available today in GC, should be challenged and definitely provide excitement in continuing the study of “basic gas chromatography” (1).
- Samples must be volatile. This is packed column thinking. Think about vapor pressure and equilibrium theory; only a few mmHg of vapor pressure is necessary to drive an analyte through a capillary column and the columns can be heated to more than 300 ˚C.
- For non-volatile and polar samples, derivatization is required. This is packed column thinking. With inert columns and inert inlets, the active sites that would adsorb polar analytes are much less common. Many analytes that once required derivatization no longer do.
- Injecting water kills columns. This is packed column thinking. Inert, chemically bonded stationary phases are much more resistant to polar solvents such as water. The more important challenge is solvent effects related to the inlet.
- LC-MS is more sensitive. This is packed column thinking. With careful consideration of sample preparation and injection chemistry, GC-MS based techniques can generate and surpass LC-MS sensitivity. And, we get classical electron ionization mass spectra.
- Use a polar column to separate polar analytes. This is packed column thinking. Highly efficient capillary columns and selective detectors, such as mass spectrometry, place much less pressure on tuning the column chemistry (selectivity) to the analytes. In my lab, we almost exclusively use 5 percent phenyl PDMS capillary columns, which greatly simplifies column management.
- Inject 1 µl of a liquid sample. This is packed column thinking. We have all been doing this since our first injection. There are myriad online sampling and injection techniques available. Split and splitless injection can severely limit the overall capability of capillary GC for trace analysis. Programmed temperature vaporization (PTV) injection has not yet become very popular although if sensitivity is the goal, it is clearly the method of choice.
In your own research and method development, I encourage you to challenge these and other common comments you may have heard about GC. The six dimensions of chemistry: sampling, sample preparation, injection, first dimension column, second dimension column (if GC×GC) and detection, that we can bring to bear on any problem will lead you to a pleasant surprise.
- HM McNair, Basic Gas Chromatography, Palo Alto: Varian, 1965 (several editions); HM McNair, JM Miller, Basic Gas Chromatography, 2nd Edition, New York: John Wiley and Sons, 2009.
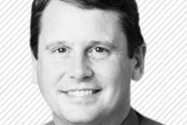
Nick is professor of Chemistry and Biochemistry and director of the Center for Academic Industry Partnership at Seton Hall University, South Orange, New Jersey, USA. He teaches advanced undergraduate and graduate courses in analytical chemistry and separation science. He has been recognized twice by the Seton Hall University Board of Regents for outstanding teaching and service to students. He maintains an active research group with projects involving rapid separations of complex mixtures, multidimensional separations, sampling techniques for chromatography, gas chromatography, and gas chromatography/mass spectrometry. He is especially interested in working with industrial and private partners in solving difficult analytical problems.