SPME Goes Mainstream
Solid-phase microextraction combines fast and simple analyte extraction with detection in the parts per trillion range. Here, the inventors of the technology predict that it is set to break out of the bioanalytical lab and into the clinic.
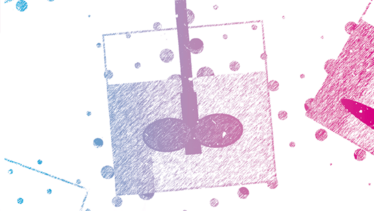
The development of solid-phase microextraction (SPME) applications began almost 25 years ago (see solid-phase microextraction 101, opposite, for an explanation of the technique) and has slowly expanded in reach.
In the early days, the primary application was in gas chromatography (GC) platforms to analyze volatile compounds. This established SPME as one of the standard methods for air analysis, and these environmental credentials were enhanced by water analysis, which today includes both spot and passive water sampling and in vivo fish sampling (3). One example of the utility of SPME is that, previously, monitoring environmental exposure and calculating bioaccumulation required that fish be sacrificed for sample collection, tissue homogenization and extraction with organic solvents. Using SPME, we simply place a small fiber in the muscle for a few minutes to extract analytes. The fiber is then removed for analysis and the fish is released. This low-invasive, non-lethal approach generates the same data as standard procedures.
The development of solid-phase microextraction (SPME) applications began almost 25 years ago (see solid-phase microextraction 101, opposite, for an explanation of the technique) and has slowly expanded in reach.
In the early days, the primary application was in gas chromatography (GC) platforms to analyze volatile compounds. This established SPME as one of the standard methods for air analysis, and these environmental credentials were enhanced by water analysis, which today includes both spot and passive water sampling and in vivo fish sampling (3). One example of the utility of SPME is that, previously, monitoring environmental exposure and calculating bioaccumulation required that fish be sacrificed for sample collection, tissue homogenization and extraction with organic solvents. Using SPME, we simply place a small fiber in the muscle for a few minutes to extract analytes. The fiber is then removed for analysis and the fish is released. This low-invasive, non-lethal approach generates the same data as standard procedures.
Another established application for SPME is in the food industry. The analysis of volatile compounds can profile trace contaminants, monitor ingredients and identify the sample’s origin. GC platforms dominate these analyses, and the attraction of combining these with SPME, and in particular headspace SPME, lies in the simplicity of the method and the availability of automated SPME autosamplers, which save time and increase precision. SPME is increasingly being coupled with liquid chromatography (LC) analysis as the range of commercially available coatings compatible with LC solvents expands (see 'Where SPME Wins' and Table 1).
More recently, attention has turned to biomedical applications, such as the monitoring of drug concentration or screening of metabolites. The development of high-resolution mass spectrometers combined with the microextraction capabilities of SPME makes SPME-MS a powerful analytical tool.
Solid-phase Microextraction 101
Solid-phase extraction (SPE) separates compounds in a mobile phase by their affinity for a particular solid in the stationary phase. In solid-phase microextraction (SPME), the polymeric sorbent (the extraction phase) can be solid or rubbery and is attached to various solid support structures (see Figure 1). Fibers, the most commonly used form of solid support, are typically used to extract volatile compounds for GC applications and non-volatiles for LC. Other SPME preparations include thin films, coated membranes, coated vessels and suspended particles (1). Most recently, SPME has been used as coated mash to improve sample clean-up; this improves sensitivity signals for a target analyte when coupled directly to a Direct Analysis in Real Time (DART)–MS/MS platform (2).
SPME relies on the diffusion of analytes from the sample matrix to the extraction phase with the goal of reaching equilibrium between phases (see Figure 2). In extractions from complex biological matrices, that is, biofluids and tissues, the biological system itself acts as a ‘buffer’ for the extraction phase, preventing saturation of the coating and competition between hydrophilic and hydrophobic analytes. In simple terms, the free-fraction concentration of polar compounds is high in aqueous biological systems, whereas non-polar species tend to be bound in complexes with macromolecules like proteins. Another important factor that contributes to balanced coverage of analytes is the affinity between the extraction phase and the compound. SPME coatings are mainly hydrophobic and therefore have high affinity for non-polar compounds and low affinity for more abundant hydrophilic species.
Clinical growth
Our group now has a significant focus on preclinical and clinical applications of SPME, spanning therapeutic drug monitoring to pharmacokinetics, and global screening of the metabolome to capturing the characteristic changes induced by stimuli, such as disease state or response to therapy.
Thanks to a collaboration with physicians at Toronto General Hospital (TGH), Canada, we have begun to validate SPME in clinical practice. Working with the Department of Anesthesia, we are studying two groups of patients: one is undergoing various surgical procedures, the other includes patients at different stages of renal dysfunction. This is the first time that the technique has been used in relatively large-scale studies in a clinical setting; we have analyzed hundreds of samples, showcasing SPME's automated, high throughput capabilities. With samples being taken from authentic cases where our colleagues at TGH need our support to solve clinical problems, SPME was truly tested because it was not possible to cherry pick the matrix, drug or concentration to be studied. Sometimes the solutions were relatively straightforward; on other occasions we encountered previously unknown difficulties.
Recently, we have been working on the pharmacokinetic profile of tranexamic acid, an anticoagulant administered to patients undergoing heart surgery alongside a cardiopulmonary bypass. The drug is often the only anticoagulant used in such cases, and its dosing regime is based on mathematical predictions. We revealed that the concentration of the drug varies from patient-to-patient, with a higher than expected average value. This suggests that some corrections to dosing may be required (4). In parallel, we monitored changes in the metabolome profile of these patients. This helped us understand the metabolite response to the surgery and pharmacotherapy, and also uncovered some notable individual response, which is of interest in terms of personalized medicine.
A further collaboration, with a group of thoracic surgeons at TGH, focuses on in vivo profiling of the lung metabolome during organ transplantation. The aim is to identify new biomarkers. This surgical team is a world leader in novel methods of lung preservation and transplantation and we hope that the planned development of SPME-based rapid diagnostic tools will further improve their results and those of other surgical teams worldwide.
Biomedical analysis
In biomedical analysis, SPME is still being predominantly used in an academic setting. Although the number of published papers is growing, the technique appears to be too new to have become established in routine analysis.
That said, SPME can be considered to be an alternative for some current assays, particularly those that require time-consuming sample preparation, such as drugs or toxins. Another niche application is where automation is difficult and analysis throughput is low. SPME offers simple automation for both volatile and non-volatile compounds – particularly in the latter case where autosamplers for leading GC platforms offer integrated SPME systems for high throughput analysis.
The main roadblock for introduction of SPME into hospitals is that mass spectrometry instrumentation, to which it is commonly coupled, is not standard. However, the powerful combination of SPME and MS is already drawing attention in therapeutic monitoring of drugs or biomarkers, which points to a major desire of the clinician: receiving information in real time. One trend in medical diagnostics is bedside analysis in place of lab analysis, which is cost-effective and user-friendly in addition to time-saving. Chip-based, microfluidic devices have been designed to be used on site by medical personnel, but these have limitations, such ashigh detection limits, carry-over and cross-selectivity. They are useful for qualitative or semi-quantitative prescreening, but do not replace powerful analytical platforms. The field of clinical diagnostics is, therefore, crying out for innovative solutions—the more tools in the toolbox, the better the chances of effective diagnosis. Coupling SPME directly with mass spectrometers to obtain fast, reproducible and accurate results is one such tool (2, 5, 6, 7, 8, 9).
Where SPME Wins
- Improved sample clean-up versus protein precipitation (PPT) and ultrafiltration
- (UF) methods
- Open-bed format eliminates cartridge-clogging issues seen with solid-phase extraction (SPE)
- One-step extraction of hydrophilic and hydrophobic compounds versus time-consuming two-step liquid-liquid extraction (LLE)
- Easy automation for GC and LC couplings
- On-site and in vivo applications do not require pumps and tubing, in contrast to microdialysis (MD) and UF
- Non-selective and highly selective extraction, depending on sorbent
- Flexible extraction phase geometry, for example, fiber and thin film (blade) for direct immersion in vivo studies.
- Low invasiveness – no sample collection required for in vivo analysis
- Direct extraction from complex solid matrices allows monitoring of spatial distribution
Drug testing
One of our most topical challenges is a project sponsored by the World Anti-Doping Agency (WADA). We are one year into the development of high-throughput methods for the simultaneous determination of over 120 doping substances of varying chemical and physical properties and the results are very promising.
There are a number of methods to screen those substances already, but most procedures focus on a certain group of compounds with similar properties. Our objective is to achieve the requisite coverage, and satisfactory recovery, in a single analysis. To do so, we use a high-throughput automated system to perform 96 analyses simultaneously. WADA criteria state that compounds must be detected at sub-ppb or -ppt levels. To meet these, we use a thin film geometry SPME device, called a blade. To date, urine analysis has been optimized and we have started working on plasma and blood. The protocol requires minimum sample handling compared with currently-used approaches.
In light of recent news reports, including Lance Armstrong’s alleged offenses, it is safe to say that there is space for a new tool to monitor doping substances in sport. We hope that SPME can be a part of this.
Table 1: SPME versus solid-phase extraction (SPE)/protein precipitation (PPT).
Feature | SPME | SPE / PPT |
---|---|---|
Nature of extraction | “Soft”, non-exhaustive | Exhaustive |
Selectivity | Based on differences in k and/or h, d | Non-selective (PPT) or selectivity based on chemistry of sorbent (SPE) |
Sensitivity | Good; quantitative transfer to GC | Very good |
Environment | Solvent-free technique with GC; solvent-less with LC | Greater solvent consumption |
Flexibility in configuration | Flexible | Limited |
Automation | Available | Available (mainly vacuum assisted) |
Convenience in handling on site and in-vivo | Very convenient | Difficult to handle on-site; in vivo application not feasible |
Integration of sampling and sample preparation steps | Yes | No |
Free concentration determinations | Yes | No |
Allows binding, speciation studies and reaction monitoring | Yes | No; requires combination with different method (i.e., ultrafiltration) |
Calibration | Unconventional: diffusion-based coefficient or Henry constant; conventional based on sample volume | Conventional |
In vivo studies
The technique most commonly used today to sample small molecules in living systems is microdialysis (MD). There are three main differences between MD and SPME:
- Metabolite coverage. MD is usually used to monitor polar species and ions. SPME coatings have better affinity for hydrophobic and semi-hydrophobic compounds.
- Temporal resolution. MD allows constant monitoring of the dialysate content, while SPME uses sampling at regular intervals or an average concentration.
- Spatial resolution. MD can only monitor different regions by using distinct probes, each connected to separate system of pumps and tubing. In SPME, segmented fibers (10) can be used to obtain information about compound concentration in different regions of an organ, for example, striatum and cortex of the brain, in a single experiment.
Given these differences, MD and SPME are highly complementary approaches to the study of living systems.
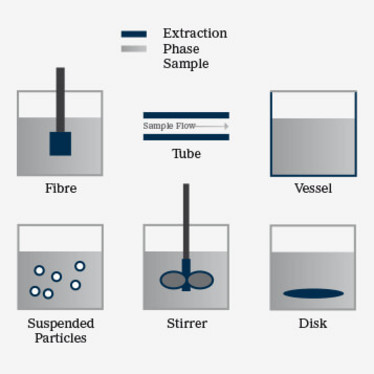
Figure 1. Various SPME solid support structures coated in extraction phase.
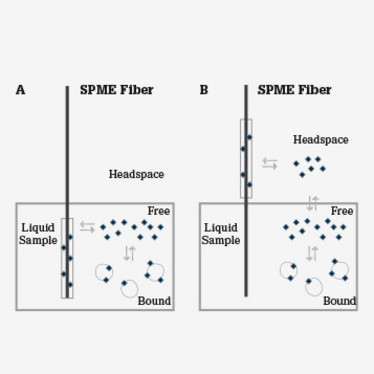
Figure 2. SPME using direct immersion (A) and headspace (B) for the determination of free concentrations.
Direct MS analysis is nothing new in analytical chemistry, but the use of complex matrices without prior sample preparation causes ionization issues and, consequently, unreliable quantitation. By integrating sampling, sample preparation and solvent-free extraction in a single step, SPME is as an excellent candidate for rapid on-site analysis. A recent illustration couples Extracted Blood Spot analysis (EBS) with DART to determine drugs in whole blood (2). The principles of EBS are similar to Dried Blood Spot analysis (DBS); a drop of blood is deposited on solid support that allows transport to an analytical laboratory. The main difference between the two approaches is the support material: for DBS, cellulose is the most common choice, while for EBS, as with SPME, a polymeric coating is used. This means that, for EBS, small molecules are extracted and introduced to the ionization source, while all interferences are removed in a quick wash step following extraction. Using this approach, we can normalize the matrix, meaning there is no significant difference between blood, plasma, urine, tissue or water. In contrast, using DBS, contaminants from the biological matrix and the support go through to the MS, affecting the quality of the data.
In vivo SPME is an alternative to EBS: first, a fast pre-equilibrium extraction (<2 min for fluids) is performed followed by a rinse step, then the fiber is placed in front of the mass spectrometer.
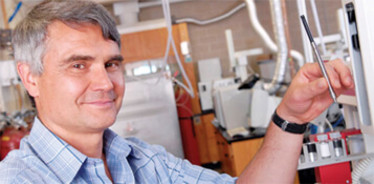
Janusz Pawliszyn: The Sweet Smell of Success
As inventor of SPME, I once received an excited call from the Caribbean. "You have made me a millionaire," said the gentleman on the other end of a static-laden line. An olfactory chemist, he thought that he had exhausted all options for finding an unstable fragrance ingredient that had disappeared from the slurry during extraction of the collected plant material. However, application of SPME recovered the lost ingredient by sampling directly from a living flower in its natural habitat and facilitated efficient transfer of the component to the analytical instrument for characterization. How that single fragrance resulted in such material wealth remains unknown to me. Perhaps he should tell his story in these pages.
The technique has certainly provided me with new experiences and research opportunities. I appreciate being recognized as an inventor and to receive innovation prizes, and it is gratifying to consult and help solve practical problems. But what is the most rewarding is when I see faster and better quality data being produced in the lab and on-site. The story of the fragrance is a good example and in the future I expect impacts in clinical and environmental applications. Indeed, that is my mission.
The promise of future SPME developments is to move away from distinct steps in sampling, sample prep, separation and detection. SPME and related technologies aid in the integration of these individual steps, constituting a paradigm shift in the field by facilitating rapid, environmentally friendly and low cost analysis.
Outlook
The uses of SPME–MS for in vivo tissue monitoring and as a rapid diagnostic tool are in the early stages of development, but the preliminary data are very promising. We believe that these are likely to be major SPME applications.
Of course, the more sophisticated the application, the greater the technological demand, so the development of new extraction phases and sampling devices must become a real focus. In terms of extraction phases, we need coatings characterized by better analyte coverage for untargeted metabolomics; molecularly imprinted polymer (MIP) sorbents for selective extractions of target analytes (drugs and biomarkers); and advances in aptamer-based phases to broaden the range of SPME applications.
From a sampling point of view, real-life situations are teaching us how SPME can be made more convenient for the user. As a result, new device designs specific to certain applications, such as tissue samplers and connections to medical devices will be developed.
Perhaps the biggest challenge is to convince not only the scientists working in hospitals and bioanalytical labs, but also those who perform clinical analysis on a daily basis about the potential of SPME. Current techniques are well established and the applied protocols are an integral part of routine analysis. Even if a new technique offers a more convenient approach or the ability to ‘tune’ results, a reliance on “tried and tested” methods is difficult to break. SPME must wait in line to be slowly accepted as a routine method unless one particular application becomes the gold standard. Perhaps pharmacokinetics or monitoring of unstable biomarkers?
- J. Pawliszyn, Handbook of Solid Phase Microextraction, Chemical Industry Press, Beijing, 2009.
- F.S. Mirnaghi and J. Pawliszyn. Reusable solid-phase microextraction coating for direct immersion whole-blood analysis and extracted blood spot sampling coupled with liquid chromatography-tandem mass spectrometry and direct analysis in real time-tandem mass spectrometry. Analytical Chemistry 84(19), 8301-9 (2012).
- O.P. Togunde et al. Optimization of solid phase microextraction for non-lethal in vivo determination of selected pharmaceuticals in fish muscle using liquid chromatography-mass spectrometry. Journal of Chromatography A. 1261, 99-106 (2012).
- B. Bojko et al , Ther. Drug Monit. 34, 31–37 (2012).
- Y. Wang, S. Nacson, J. Pawliszyn. The coupling of solid-phase microextraction/surface enhanced laser desorption/ionization to ion mobility spectrometry for drug analysis. Analitica Chimica Acta. 582(1), 50-54 (2007).
- Y. Wang et al. Solid-phase microextraction combined with surface-enhanced laser desorption/ionization introduction for ion mobility spectrometry and mass spectrometry using polypyrrole coatings. Rapid Communications in Mass Spectrometry. 18(2), 157-162 (2004).
- H. Tong et al. Solid phase microextraction with matrix assisted laser desorption/ionization introduction to mass spectrometry and ion mobility spectrometry. Analyst. 127(9), 1207-1210 (2002).
- Y. Wang et al.. High-performance SPME/AP MALDI system for high-throughput sampling and determination of peptides. Analytical Chemistry. 77(24), 8095-8101 (2005).
- S. Perera et al. Coupling solid-phase microextraction and laser desorption ionization for rapid identification of biological material. Rapid Communications in Mass Spectrometry. 26(7), 853-862 (2012).
- X . Zhang et al. Tissue-specific in vivo bioconcentration of pharmaceuticals in rainbow trout (oncorhynchus mykiss) using space-resolved solid-phase microextraction. Environmental Science & Technology 44(9), 3417-22 (2010).
Barbara Bojko is Head of Department, Faculty of Pharmacy, at Nicolaus Copernicus University in Torun, Bydgoszcz, Poland
Janusz Pawliszyn is Professor at the Department of Chemistry, University of Waterloo, Ontario, Canada.