Marking Progress
Monika Dittman (Agilent Technologies) and Fabrice Gritti (Waters) offer a manufacturer point of view on the practical limits of LC.
What is the current state of play?
Monika Dittman: In 1D-HPLC, using sub-2µm particles and (U)HPLC instruments operating at over 1000 bar, the time to reach a desired plate number has been reduced roughly by a factor of 5 (30,000 plates in four minutes with 1.8 µm particles at 1000 bar compared to 20 minutes with 5 µm particles at 300 bar) in routine analysis. The gain in performance can be used to obtain higher resolution in the same separation time (typically a factor of 3–4 on plate number or a factor of 1.5 in resolution/peak capacity), depending on the separation conditions.
These gains have enabled the fast separation of complex samples, and reduced the effort and time required for method development because a lack in selectivity is counteracted by increasing efficiency.
A further increase in operating pressure along with a further decrease in particle size could provide some additional improvement in speed and/or resolution, but 1D-HPLC eventually reaches a theoretical limit. Multidimensional LC can provide much larger peak capacities in a shorter time, which offers more power for very complex samples. And although it cannot be considered a routine technology right now, commercial solutions are available and being used by an increasing number of researchers.
Fabrice Gritti: Fast 1D-LC analyses of moderately complex samples and biomolecules is possible with short (5–10 cm) and narrow-bore (2.1 mm) columns packed with sub-2 µm particles. For the same level of efficiency, the analysis times in UHPLC have decreased by a factor of 10 relative to those observed in HPLC using 15-cm long columns packed with 5 µm particles. The volume consumption of eluent is also reduced by a factor of 10. The only downside is that system pressures have increased from 100-300 in HPLC to 400-1200 bars in UHPLC. The milestone for such an achievement was the emergence in 2004 of instrument pumps capable of delivering high flow rates at pressure of 1 kbar and the preparation of high strength sub-2 µm silica particles.
For all that, UHPLC rapidly touched its limits in terms of resolution because most standard UHPLC systems cannot provide more than 20 percent of the intrinsic efficiency of short (5 cm) and narrow-bore (2.1 mm) columns packed with sub-2 µm core-shell particles. System dispersion must be reduced further without precluding detection sensitivity (by diminishing the UV cell volume and path length) and speed (by shrinking the inner diameter of the connecting tubes). The transfer of United States Pharmacopeia methods from HPLC to UHPLC is also a critical issue because pressure and frictional heating affect retention (identification), peak width (resolution), and peak area (quantification) in UHPLC.
Since 2007, the lowest reduced plate heights of 2.1 mm to 4.6 mm i.d. analytical columns (capillary columns excluded) have been reached with core-shell packing materials (h=1.4) and 2.7 µm Halo silica particles. These developments mean there is no need for ultra-high pressures but it is still necessary to optimize standard HPLC instruments to reduce band spreading.
Resolving complex sample mixtures is routine practice using online and offline 2D (time x time) LC. Some commercial instruments provide customers with the possibility of 2D analyses, which plays an important role in polymer separation, in proteomics, and in genomics. Yet, 2D-LC faces some practical difficulties in terms of column orthogonality, solvent compatibility, sensitivity loss, and MS detection rate. For instance, the digest of host cell proteins having a dynamic range of concentration from one to more than a million cannot be solved satisfactorily by 2D-LC/MS.
Hyphenated LC/MSn techniques are now a routine analysis tool in any lab around the world. LC is still indispensable to fractionate complex mixtures, while MS enables identification of all the precursor and product ions. The limits of MS detection are quantification and the scan rate.
What contributed to these advancements in LC?
MD: Calvin Giddings laid the foundation for ultra-high pressure LC back in the 1960s when he predicted that gains in resolution/time could be achieved with higher operating pressures. Then, more than 20 years ago, James Jorgenson’s group demonstrated extremely high column efficiencies when operating with 1 µm particles at very high pressures.
In 2004, Waters introduced the first commercial system that could operate at 1000 bar, together with 1.7-µm particle packed columns, which subsequently led to every major HPLC equipment vendor offering UHPLC systems with operating pressures up to 1500 bar. Although routine analyses are still performed under standard HPLC conditions, customers often buy UHPLC systems when replacing older equipment.
FG: I agree with Monika. Giddings’ basic theory of chromatography has continuously pushed manufacturers to innovate and build new instruments (higher pressure, smaller system dispersion, 2D separation systems) and smaller particles to achieve faster separation and higher resolution performances by LC. Theory is obviously a major driving force for innovation.
The success of the sub-3 µm core-shell packing materials for analyzing small molecules is due to some unexpected properties of their beds. They are more radially uniform than those packed with conventional particles. This observation was not predicted by any theory of chromatography, which explains why most column manufacturers were initially reluctant in preparing such new materials. So, innovations can also result from pure luck! In contrast, Horvath predicted the use of core-shell particles for separating heavier molecules in the early 1960s based on the reduction of the average diffusion time across giant 50 µm particles.
In any case, technical achievements are delivering faster analyses, higher analysis throughput, and solving more complex sample mixtures than the old conventional HPLC techniques. But we must not rest on our laurels; separation science still faces numerous challenges:
- The complete resolution of protein mixtures in host cells for which the dynamic range of concentration extends from 1 to 107. This requires better isolation of the least abundant from the most concentrated proteins, more accurate quantification of the detected ions, and higher MS scan rates.
- The separation of glycan-binding protein for determining biological mechanisms.
- The separation of protein isoforms that differ by a few mutations of amino acids.
- A need to increase analysis throughput of chiral candidates during the drug discovery process demanded by pharmaceutical companies.
Where are we heading?
FG: Computerized molecular dynamics studies will further refine our established, basic theories of chromatography (Schure, Tallarek, and Mountain all talk about this). These studies enable a deeper understanding of the mass transfer and retention processes in LC from a molecular scale. In the end, they will consolidate or correct our old theories, and, in practice, they will enable the new design of the structures (kinetics or mass transfer) and of the chemistries (thermodynamics or selectivity) of separation systems for targeted performance.
UHPLC systems (injection, tubes, connections, detection) and column hardware (end-fittings + frits) will need redesigning to benefit fully from short (< 5cm) narrow-bore (1-2.1 mm i.d.) columns packed with 0.5 µm particles for analyzing biomolecules at low linear velocity.
Sub-1 µm particles are currently useless for the analysis of small molecules: that said, UHPLC systems would have to withstand pressures as high as 3 kbar to deliver optimum velocity. This could become a reality in years to come. Ideally, on-column injection/detection is required to eliminate system bandspreading. Ultra-low small injection volume (< 10 nL), short and small i.d. connecting tubes (< 50 µm), small volume detection cell (< 100 nL) or on-tube detection, and improved column end-fittings need developing. Currently, we are just reaching the limits of miniaturizing “classical” UHPLC parts, operating in a range of flow rate from 0.1 to 1.0 mL/min with today’s technology.
We must push research efforts for fully integrated LC-MS separation systems using either packed micro-channels or capillary tubes (100-500 µm i.d.) as separation media. The challenge with small volume separation systems is the detection sensitivity (too short path length). New detection techniques other than UV-Vis are therefore needed. Light emitting diodes are a solution (excellent monochromaticity and noiseless signal), yet they cannot cover a wide continuous range of wavelengths. In terms of speed, a larger number of compounds will be identified if the scan time of MS detectors is reduced. Injection, separation, and MS detection devices should be integrated to minimize losses of resolution and sensitivity.
What are the limits?
MD: With modern UHPLC instruments and small particles we have come very close to the limits that can theoretically be reached in 1D LC. However, very important improvements would include ease-of-use and maintenance, cost of ownership, overall system intelligence – those things that ultimately improve efficiency and reduce cost. With multidimensional LC, the complexity of instrumentation and applications had been limiting its extended use, but this is starting to change.
FG: The current limiting factors of UHPLC techniques in terms of resolution and speed are essentially instrumental: the column hardware affects the resolution power of short columns severely for the least retained compounds, small UV cells are reducing sensitivity, and very narrow connecting tubes generate too much backpressure for the current generation of pumps.
What other technologies/techniques will affect LC?
MD: We should expect to see MS-systems that keep pace with fast separations. In addition, we need intelligent software to not only generate data but also enable their meaningful use – one of the current bottlenecks is efficient and fast handling of data produced by ultra-fast, ultra-high resolution analysis.
Where do you expect LC to be in five years?
MD: MS will become more of a routine detection method (as in GC already) and multidimensional LC will become standard for medium complex samples. In addition, multidimensional LC-MS (or even multidimensional LC-ion mobility spectrometry-MS/MS) will become standard for complex samples.
As the majority of LC users will not be separation scientists but organic chemists or biologists the big challenge will be to create intelligent software solutions that support users in instrument operation, method development and data interpretation. This will be of particular importance for the success of multi-dimensional LC to be adopted as a routine technique.
FG: In five years from now, we should expect that UHPLC coupled to MS detection will finally play a major role in most academic and industrial laboratories. The high price of UHPLC technology, the difficulty to transfer well-established methods developed for HPLC to new methods for UHPLC, and the need for integrated MS detectors (everyone needs a LC-MS detector now, not a simple LC-UV instrument) holds back progress in the instrument market. SFC-based instrumentations (SFC-MS, LC-SFC-MS or SFC-LC-MS) will become available and friendly to use given the numerous advantages of supercritical CO2 fluids (cheap and green solvent, fast mass transfer and high resolution, high analysis speed).
The boom in micro-fluidic devices will likely provide a new generation of high-resolution portable instrumentation for routine analysis. Nano-LC/MS at very high pressures should enable the use of relatively short capillary columns or micro-channels packed with sub-1 µm particles for the analysis of biological samples.
If we look further to 10 years, we may see the emergence of 3D-printing technologies capable of reproducing perfectly ordered column structures with a characteristic dimension smaller than a few µm. Fabricated from relatively cheap raw silicon material, these printed columns could well supplant the current silica-based particulate and monolith technologies.
Looking forward even further, the ultimate and unified analytical tool, which could solve any of the analysts’ needs will feature in a single apparatus selected extraction units (by either liquid or supercritical fluids), selected injection devices (head space for GC analysis of volatiles, trap beds for SFC-based extracts, standard LC injectors), selected pump devices (for GC, SFC, or LC separations), switch-column valves (for 2D analyses), and MSn detection. All these technologies are currently available but as separate units for most. The fundamental, engineering, and technical challenges required to bring them altogether are obviously immense but not out of reach in the long term. At least, research efforts towards this direction should be funded and encouraged.
From an academic viewpoint, separation scientists are becoming a rare species even close to extinction after the passing of Giddings, Horvath, and Guiochon. Chromatography is no longer considered as an indispensable science instead is seen as merely a preparative tool. Yet, it should be remembered that most improvements made in GC and LC over the last 50 years were based on sound physical chemistry, material chemistry, and engineering. Future challenges in separation sciences and their new technologies to come will not escape this rule.
Expanding LC Boundaries
By Mary J. Wirth
Have We Really Peaked?
By James Jorgenson
Marching Ever Onward
By Gert Desmet
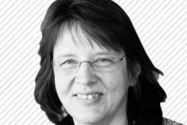
In 1988, after finishing her PhD and spending two years as a post-doc at UC Berkeley, USA, Monika Dittmann joined HP (now Agilent Technologies) as an R&D scientist. In her career with HP/Agilent she has worked on the development of instruments and technologies in the fields of HPLC, Capillary Electrophoresis, Capillary Electro-Chromatography and Micro Fluidics as a research scientist and project manager. Monika currently holds the position of a Principal Scientist working on the design and development of next generation HPLC systems.
Fabrice Gritti is based at the Waters Corporation, Milford, USA.